Osteopontin (OPN)
Osteopontin (OPN), also known as bone /sialoprotein I (BSP-1 or BNSP), early T-lymphocyte activation (ETA-1), secreted phosphoprotein 1 (SPP1), 2ar and Rickettsia resistance (Ric), is a protein that in humans is encoded by the SPP1 gene (secreted phosphoprotein 1). The murine ortholog is Spp1. Osteopontin is a SIBLING (glycoprotein) that was first identified in 1986 in osteoblasts.
The family of non-collagenous proteins known as SIBLING proteins, standing for small integrin-binding ligand, N-linked glycoprotein, are components of the extracellular matrix of bone and dentin. Evidence shows that these proteins play key roles in the mineralization of these tissues.
The following are categorized as SIBLING proteins:
- osteopontin (OPN)
- bone sialoprotein (BSP)
- Bone sialoprotein (BSP) is a component of mineralized tissues such as bone, dentin, cementum and calcified cartilage. BSP is a significant component of the bone extracellular matrix and has been suggested to constitute approximately 8% of all non-collagenous proteins found in bone and cementum. BSP, a SIBLING protein, was originally isolated from bovine cortical bone as a 23-kDa glycopeptide with high sialic acid content.
- The human variant of BSP is called bone sialoprotein 2 also known as cell-binding sialoprotein or integrin-binding sialoprotein and is encoded by the IBSP gene.
- Secondary structure prediction and hydrophobicity analyses suggest that the primary sequence of BSP has an open, flexible structure with the potential to form regions of α-helix and some β-sheet. However, the majority of studies have demonstrated that BSP has no α-helical or β-sheet structure by 1D NMR and circular dichroism. Analysis of native protein by electron microscopy confirm that the protein has an extended structure approximately 40 nm in length. This flexible conformation suggests that the protein has few structural domains, however it has been suggested that there may be several spatially segmented functional domains including a hydrophobic collagen-binding domain (rattus norvegicus residues 36–57), a hydroxyapatite-nucleating region of contiguous glutamic acid residues (rattus norvegicus residues 78–85, 155–164) and a classical integrin-binding motif (RGD) near the C-terminal (rattus norvegicus residues 288–291).
- BSP has been demonstrated to be extensively post-translationally modified, with carbohydrates and other modifications comprising approximately 50% of the molecular weight of the native protein. These modifications, which include N- and O-linked glycosylation, tyrosine sulfation and serine and threonine phosphorylation, make the protein highly heterogeneous.
- The amount of BSP in bone and dentin is roughly equal, however the function of BSP in these mineralized tissues is not known. One possibility is that BSP acts as a nucleus for the formation of the first apatite crystals. As the apatite forms along the collagen fibres within the extracellular matrix, BSP could then help direct, redirect or inhibit the crystal growth.
- Additional roles of BSP are angiogenesis and protection from complement-mediated cell lysis. Regulation of the BSP gene is important to bone matrix mineralization and tumor growth in bone.
- dentin matrix protein 1 (DMP1)
- Dentin matrix acidic phosphoprotein 1 is a protein that in humans is encoded by the DMP1 gene.
- Dentin matrix acidic phosphoprotein is an extracellular matrix protein and a member of the small integrin-binding ligand N-linked glycoprotein (SIBLING) family (other members being DSPP, IBSP, MEPE, and SPP1). This protein, which is critical for proper mineralization of bone and dentin, is present in diverse cells of bone and tooth tissues. The protein contains a large number of acidic domains, multiple phosphorylation sites, a functional arg-gly-asp cell attachment sequence, and a DNA binding domain. In undifferentiated osteoblasts it is primarily a nuclear protein that regulates the expression of osteoblast-specific genes. During osteoblast maturation the protein becomes phosphorylated and is exported to the extracellular matrix, where it orchestrates mineralized matrix formation. Mutations in the gene are known to cause autosomal recessive hypophosphatemia, a disease that manifests as rickets and osteomalacia. The gene structure is conserved in mammals. Two transcript variants encoding different isoforms have been described for this gene.
- dentin sialophosphoprotein (DSPP)
- Dentin sialophosphoprotein is a precursor protein for other proteins found in the teeth. It is produced by cells (odontoblasts) inside the teeth (dental pulp), and in smaller quantities by bone tissues (osteoblasts and osteocytes). It is required for normal hardening (mineralisation) of teeth. During teeth development, it is broken down into three proteins such as dentin sialoprotein (DSP), dentin glycoprotein (DGP), and dentin phosphoprotein (DPP). These proteins become the major non-collagenous components of teeth. Their distribution in the collagen matrix of the forming dentin suggests these proteins play an important role in the regulation of mineral deposition. Additional evidence for this correlation is phenotypically manifested in patients with mutant forms of dentin sialophosphoprotein. Such patients suffer dental anomalies including type III dentinogenesis imperfecta.
- It is coded by a gene of the same name (dentin sialophosphoprotein, or DSPP) present on human chromosome 4. This gene encodes two principal proteins of the dentin extracellular matrix of the tooth. The preproprotein is secreted by odontoblasts and cleaved into dentin sialoprotein and dentin phosphoprotein. Dentin phosphoprotein is thought to be involved in the biomineralization process of dentin. Mutations in this gene have been associated with dentinogenesis imperfecta-1; in some individuals, dentinogenesis imperfecta occurs in combination with an autosomal dominant form of deafness. Allelic differences due to repeat polymorphisms have been found for this gene.
- matrix extracellular phosphoglycoprotein (MEPE)
The genes coding for members of the SIBLING protein family are similarly organized and are all located on human chromosome 4q21-23.
- Qin, C.; Baba, O.; Butler, W.T. (2004). “Post-Translational Modifications of Sibling Proteins and Their Roles in Osteogenesis and Dentinogenesis”. Critical Reviews in Oral Biology & Medicine. 15 (3): 126–36. doi:10.1177/154411130401500302. PMID 15187031.
- Chaplet, M; De Leval, L; Waltregny, D; Detry, C; Fornaciari, G; Bevilacqua, G; Fisher, LW; Castronovo, V; Bellahcène, A (2003). “Dentin Matrix Protein 1 is Expressed in Human Lung Cancer”. Journal of Bone and Mineral Research. 18 (8): 1506–12. doi:10.1359/jbmr.2003.18.8.1506. PMID 12929940. S2CID 24870810. INIST 15005341.
- Rittling, Susan R.; Denhardt, David T. (1999). “Osteopontin Function in Pathology: Lessons from Osteopontin-Deficient Mice”. Nephron Experimental Nephrology. 7 (2): 103–13. doi:10.1159/000020591. PMID 10213864. S2CID 19785030.
The prefix osteo- indicates that the protein is expressed in bone, although it is also expressed in other tissues. The suffix -pontin is derived from “pons,” the Latin word for bridge, and signifies osteopontin’s role as a linking protein. Osteopontin is an extracellular structural protein and therefore an organic component of bone.
The gene has 7 exons, spans 5 kilobases in length and in humans it is located on the long arm of chromosome 4 region 22 (4q1322.1). The protein is composed of ~300 amino acids residues and has ~30 carbohydrate residues attached, including 10 sialic acid residues, which are attached to the protein during post-translational modification in the Golgi apparatus. The protein is rich in acidic residues: 30-36% are either aspartic or glutamic acid.
Structure
OPN is a highly negatively charged, heavily phosphorylated extracellular matrix protein that lacks an extensive secondary structure as an intrinsically disordered protein. It is composed of about 300 amino acids (297 in mouse; 314 in human) and is expressed as a 33-kDa nascent protein; there are also functionally important cleavage sites. OPN can go through posttranslational modifications, which increase its apparent molecular weight to about 44 kDa. The OPN gene is composed of 7 exons, 6 of which containing coding sequence. The first two exons contain the 5′ untranslated region (5′ UTR). Exons 2, 3, 4, 5, 6, and 7 code for 17, 13, 27, 14, 108 and 134 amino acids, respectively. All intron-exon boundaries are of the phase 0 type, thus alternative exon splicing maintains the reading frame of the OPN gene.
- Kalmar L, Homola D, Varga G, Tompa P (September 2012). “Structural disorder in proteins brings order to crystal growth in biomineralization”. Bone. 51 (3): 528–534. doi:10.1016/j.bone.2012.05.009. PMID 22634174.
- Wang KX, Denhardt DT (2008). “Osteopontin: role in immune regulation and stress responses”. Cytokine & Growth Factor Reviews. 19 (5–6): 333–345. doi:10.1016/j.cytogfr.2008.08.001. PMID 18952487.
- Rangaswami H, Bulbule A, Kundu GC (February 2006). “Osteopontin: role in cell signaling and cancer progression”. Trends in Cell Biology. 16 (2): 79–87. doi:10.1016/j.tcb.2005.12.005. PMID 16406521.
- Young MF, Kerr JM, Termine JD, Wewer UM, Wang MG, McBride OW, Fisher LW (August 1990). “cDNA cloning, mRNA distribution and heterogeneity, chromosomal location, and RFLP analysis of human osteopontin (OPN)”. Genomics. 7 (4): 491–502. doi:10.1016/0888-7543(90)90191-V. PMID 1974876.
- Kiefer MC, Bauer DM, Barr PJ (April 1989). “The cDNA and derived amino acid sequence for human osteopontin”. Nucleic Acids Research. 17 (8): 3306. doi:10.1093/nar/17.8.3306. PMC 317745. PMID 2726470.
- Crosby AH, Edwards SJ, Murray JC, Dixon MJ (May 1995). “Genomic organization of the human osteopontin gene: exclusion of the locus from a causative role in the pathogenesis of dentinogenesis imperfecta type II”. Genomics. 27 (1): 155–160. doi:10.1006/geno.1995.1018. PMID 7665163.
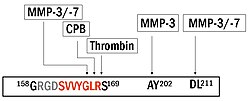
Isoforms
Full-length OPN (OPN-FL) can be modified by thrombin cleavage, which exposes a cryptic sequence, SVVYGLR on the cleaved form of the protein known as OPN-R (Fig. 1). This thrombin-cleaved OPN (OPN-R) exposes an epitope for integrin receptors of α4β1, α9β1, and α9β4. These integrin receptors are present on a number of immune cells such as mast cells, neutrophils, and T cells. It is also expressed by monocytes and macrophages. Upon binding these receptors, cells use several signal transduction pathways to elicit immune responses in these cells. OPN-R can be further cleaved by Carboxypeptidase B (CPB) by removal of C-terminal arginine and become OPN-L. The function of OPN-L is largely unknown.
- Laffón A, García-Vicuña R, Humbría A, Postigo AA, Corbí AL, de Landázuri MO, Sánchez-Madrid F (August 1991). “Upregulated expression and function of VLA-4 fibronectin receptors on human activated T cells in rheumatoid arthritis”. The Journal of Clinical Investigation. 88 (2): 546–552. doi:10.1172/JCI115338. PMC 295383. PMID 1830891.
- Seiffge D (December 1996). “Protective effects of monoclonal antibody to VLA-4 on leukocyte adhesion and course of disease in adjuvant arthritis in rats”. The Journal of Rheumatology. 23 (12): 2086–2091. PMID 8970045.
- Reinholt FP, Hultenby K, Oldberg A, Heinegård D (June 1990). “Osteopontin–a possible anchor of osteoclasts to bone”. Proceedings of the National Academy of Sciences of the United States of America. 87 (12): 4473–4475. Bibcode:1990PNAS…87.4473R. doi:10.1073/pnas.87.12.4473. PMC 54137. PMID 1693772.
- Banerjee A, Apte UM, Smith R, Ramaiah SK (March 2006). “Higher neutrophil infiltration mediated by osteopontin is a likely contributing factor to the increased susceptibility of females to alcoholic liver disease”. The Journal of Pathology. 208 (4): 473–485. doi:10.1002/path.1917. PMID 16440289. S2CID 45879742.
- Sodek J, Batista Da Silva AP, Zohar R (May 2006). “Osteopontin and mucosal protection”. Journal of Dental Research. 85 (5): 404–415. doi:10.1177/154405910608500503. PMID 16632752. S2CID 25898774.[permanent dead link
It appears an intracellular variant of OPN (iOPN) is involved in a number of cellular processes including migration, fusion and motility. Intracellular OPN is generated using an alternative translation start site on the same mRNA species used to generate the extracellular isoform. This alternative translation start site is downstream of the N-terminal endoplasmic reticulum-targeting signal sequence, thus allowing cytoplasmic translation of OPN.
- Zohar R, Suzuki N, Suzuki K, Arora P, Glogauer M, McCulloch CA, Sodek J (July 2000). “Intracellular osteopontin is an integral component of the CD44-ERM complex involved in cell migration”. Journal of Cellular Physiology. 184 (1): 118–130. doi:10.1002/(SICI)1097-4652(200007)184:1<118::AID-JCP13>3.0.CO;2-Y. PMID 10825241. S2CID 11548419.
- Suzuki K, Zhu B, Rittling SR, Denhardt DT, Goldberg HA, McCulloch CA, Sodek J (August 2002). “Colocalization of intracellular osteopontin with CD44 is associated with migration, cell fusion, and resorption in osteoclasts”. Journal of Bone and Mineral Research. 17 (8): 1486–1497. doi:10.1359/jbmr.2002.17.8.1486. PMID 12162503. S2CID 21307088.
- Zhu B, Suzuki K, Goldberg HA, Rittling SR, Denhardt DT, McCulloch CA, Sodek J (January 2004). “Osteopontin modulates CD44-dependent chemotaxis of peritoneal macrophages through G-protein-coupled receptors: evidence of a role for an intracellular form of osteopontin”. Journal of Cellular Physiology. 198 (1): 155–167. doi:10.1002/jcp.10394. PMID 14584055. S2CID 21075107.
- Junaid A, Moon MC, Harding GE, Zahradka P (February 2007). “Osteopontin localizes to the nucleus of 293 cells and associates with polo-like kinase-1”. American Journal of Physiology. Cell Physiology. 292 (2): C919–C926. doi:10.1152/ajpcell.00477.2006. PMID 17005603.
- Shinohara ML, Kim HJ, Kim JH, Garcia VA, Cantor H (May 2008). “Alternative translation of osteopontin generates intracellular and secreted isoforms that mediate distinct biological activities in dendritic cells”. Proceedings of the National Academy of Sciences of the United States of America. 105 (20): 7235–7239. Bibcode:2008PNAS..105.7235S. doi:10.1073/pnas.0802301105. PMC 2438233. PMID 18480255
Various human cancers, including breast cancer, have been observed to express splice variants of OPN. The cancer-specific splice variants are osteopontin-a, osteopontin-b, and osteopontin-c. Exon 5 is lacking from osteopontin-b, whereas osteopontin-c lacks exon 4. Osteopontin-c has been suggested to facilitate the anchorage-independent phenotype of some human breast cancer cells due to its inability to associate with the extracellular matrix.
- He B, Mirza M, Weber GF (April 2006). “An osteopontin splice variant induces anchorage independence in human breast cancer cells”. Oncogene. 25 (15): 2192–2202. doi:10.1038/sj.onc.1209248. PMID 16288209. S2CID 6632395.
- Mirza M, Shaughnessy E, Hurley JK, Vanpatten KA, Pestano GA, He B, Weber GF (February 2008). “Osteopontin-c is a selective marker of breast cancer”. International Journal of Cancer. 122 (4): 889–897. doi:10.1002/ijc.23204. PMID 17960616. S2CID 22146646.
Tissue distribution
Osteopontin is expressed in a variety of tissue types including cardiac fibroblasts, preosteoblasts, osteoblasts, osteocytes, odontoblasts, some bone marrow cells, hypertrophic chondrocytes, dendritic cells, macrophages, smooth muscle, skeletal muscle myoblasts, endothelial cells, and extraosseous (non-bone) cells in the inner ear, brain, kidney, deciduum, and placenta. Synthesis of osteopontin is stimulated by calcitriol (1,25-dihydroxy-vitamin D3).
- Ashizawa N, Graf K, Do YS, Nunohiro T, Giachelli CM, Meehan WP, et al. (November 1996). “Osteopontin is produced by rat cardiac fibroblasts and mediates A(II)-induced DNA synthesis and collagen gel contraction”. The Journal of Clinical Investigation. 98 (10): 2218–2227. doi:10.1172/JCI119031. PMC 507670. PMID 8941637.
- Murry CE, Giachelli CM, Schwartz SM, Vracko R (December 1994). “Macrophages express osteopontin during repair of myocardial necrosis”. The American Journal of Pathology. 145 (6): 1450–1462. PMC 1887495. PMID 7992848.
- Ikeda T, Shirasawa T, Esaki Y, Yoshiki S, Hirokawa K (December 1993). “Osteopontin mRNA is expressed by smooth muscle-derived foam cells in human atherosclerotic lesions of the aorta”. The Journal of Clinical Investigation. 92 (6): 2814–2820. doi:10.1172/JCI116901. PMC 288482. PMID 8254036.
- Uaesoontrachoon K, Yoo HJ, Tudor EM, Pike RN, Mackie EJ, Pagel CN (April 2008). “Osteopontin and skeletal muscle myoblasts: association with muscle regeneration and regulation of myoblast function in vitro”. The International Journal of Biochemistry & Cell Biology. 40 (10): 2303–2314. doi:10.1016/j.biocel.2008.03.020. PMID 18490187.
Regulation
Regulation of the osteopontin gene expression is incompletely understood. Different cell types may differ in their regulatory mechanisms of the OPN gene. OPN expression in bone predominantly occurs by osteoblasts and osteocyctes (bone-forming cells) as well as osteoclasts (bone-resorbing cells). Runx2 (aka Cbfa1) and osterix (Osx) transcription factors are required for the expression of OPN Runx2 and Osx bind promoters of osteoblast-specific genes such as Col1α1, Bsp, and Opn and upregulate transcription.
- Merry K, Dodds R, Littlewood A, Gowen M (April 1993). “Expression of osteopontin mRNA by osteoclasts and osteoblasts in modelling adult human bone”. Journal of Cell Science. 104 (4): 1013–1020. doi:10.1242/jcs.104.4.1013. PMID 8314886.
- Nakashima K, Zhou X, Kunkel G, Zhang Z, Deng JM, Behringer RR, de Crombrugghe B (January 2002). “The novel zinc finger-containing transcription factor osterix is required for osteoblast differentiation and bone formation”. Cell. 108 (1): 17–29. doi:10.1016/S0092-8674(01)00622-5. PMID 11792318. S2CID 14030684.
- Ducy P, Zhang R, Geoffroy V, Ridall AL, Karsenty G (May 1997). “Osf2/Cbfa1: a transcriptional activator of osteoblast differentiation”. Cell. 89 (5): 747–754. doi:10.1016/S0092-8674(00)80257-3. PMID 9182762. S2CID 16979723.
Hypocalcemia and hypophosphatemia (instances that stimulate kidney proximal tubule cells to produce calcitriol (1α,25-dihydroxyvitamin D3)) lead to increases in OPN transcription, translation and secretion. This is due to the presence of a high-specificity vitamin D response element (VDRE) in the OPN gene promoter.
- Yucha C, Guthrie D (December 2003). “Renal homeostasis of calcium”. Nephrology Nursing Journal. 30 (6): 755–764. PMID 14730782.
- Prince CW, Butler WT (September 1987). “1,25-Dihydroxyvitamin D3 regulates the biosynthesis of osteopontin, a bone-derived cell attachment protein, in clonal osteoblast-like osteosarcoma cells”. Collagen and Related Research. 7 (4): 305–313. doi:10.1016/s0174-173x(87)80036-5. PMID 3478171.
- Oldberg A, Jirskog-Hed B, Axelsson S, Heinegård D (December 1989). “Regulation of bone sialoprotein mRNA by steroid hormones”. The Journal of Cell Biology. 109 (6 Pt 1): 3183–3186. doi:10.1083/jcb.109.6.3183. PMC 2115918. PMID 2592421.
- Chang PL, Prince CW (April 1991). “1 alpha,25-dihydroxyvitamin D3 stimulates synthesis and secretion of nonphosphorylated osteopontin (secreted phosphoprotein 1) in mouse JB6 epidermal cells”. Cancer Research. 51 (8): 2144–2150. PMID 2009532
Osteopontin (OPN) expression is modulated by Schistosoma mansoni egg antigen.
- Pereira TA, Vaz de Melo Trindade G, Trindade Santos E, Pereira FE, Souza MM (May 2021). “Praziquantel pharmacotherapy reduces systemic osteopontin levels and liver collagen content in murine schistosomiasis mansoni”. International Journal for Parasitology. 51 (6): 437–440. doi:10.1016/j.ijpara.2020.11.002. PMID 33493521. S2CID 231711719.
Schistosoma mansoni egg antigens directly stimulate the expression of the profibrogenic molecule osteopontin (OPN), and systemic OPN levels strongly correlate with disease severity, suggesting its use as a potential morbidity biomarker. Investigation into the impact of Praziquantel use on systemic OPN levels and on liver collagen deposition in chronic murine schistosomiasis revealed that Praziquantel treatment significantly reduced systemic OPN levels and liver collagen deposition, indicating that OPN could be a reliable tool for monitoring PZQ efficacy and fibrosis regression.
- Pereira TA, Vaz de Melo Trindade G, Trindade Santos E, Pereira FE, Souza MM (May 2021). “Praziquantel pharmacotherapy reduces systemic osteopontin levels and liver collagen content in murine schistosomiasis mansoni”. International Journal for Parasitology. 51 (6): 437–440. doi:10.1016/j.ijpara.2020.11.002. PMID 33493521. S2CID 231711719.
- “Editorial”. International Journal for Parasitology. 26 (8–9): 797–798. 1996-08-09. doi:10.1016/0020-7519(96)00065-3. ISSN 0020-7519.
A regenerative immunotherapy approach using natural helminth derivatives is described as using soluble egg antigen (SEA) to stimulate production of interleukin (IL)-4 and other type 2-associated cytokines without negative infection-related sequelae. The regenerative SEA (rSEA) applied to a murine muscle injury induced accumulation of IL-4-expressing T helper cells, eosinophils, and regulatory T cells and decreased expression of IL-17A in gamma delta (γδ) T cells, resulting in improved repair and decreased fibrosis. Encapsulation and controlled release of rSEA in a hydrogel further enhanced type 2 immunity and larger volumes of tissue repair. The broad regenerative capacity of rSEA was validated in articular joint and corneal injury models.
- David R. Maestas Jr. Helminth egg derivatives as proregenerative immunotherapies PNAS Vol. 120 | No. 8 APPLIED BIOLOGICAL SCIENCES February 13, 2023 doi.org/10.1073/pnas.2211703120
One of the most widely used antigenic preparations from schistosomes is SEA (soluble egg antigen). The crude extract obtained from the mature eggs is isolated from the tissues of the definitive host. The use of SEA has been critical in dissecting immunologically-driven responses to the eggs in an active infection. Techniques for SEA isolation were first described by Boros and Warren (1970). As with any crude extract of a multicellular organism, it consists of a bewildering array of components, such as proteins, glycoproteins, polysaccharides and glycolipids.
- Boros DL, Warren KS. Delayed hypersensitivity-type granuloma formation and dermal reaction induced and elicited by a soluble factor isolated from Schistosoma mansoni eggs. J Exp Med. 1970 Sep 1;132(3):488-507. doi: 10.1084/jem.132.3.488. PMID: 5535626; PMCID: PMC2138804.
- Tucker, M. S., Karunaratne, L. B., Lewis, F. A., Frietas, T. C., and Liang, Y-S. 2013. Schistosomiasis, in Current Protocols in Immunology 19.1.1-19.1.57, John Wiley and Sons, Inc., (R. Coico, Ed). Published online November 2013 in Wiley Online Library (wileyonlinelibrary.com). doi: 10.1002/0471142735.im1901s103.
Schistosoma mansoni is a water-borne parasite of humans, and belongs to the group of blood flukes (Schistosoma). The adult lives in the blood vessels (mesenteric veins) near the human intestine. It causes intestinal schistosomiasis (similar to S. japonicum, S. mekongi, S. guineensis, and S. intercalatum). Clinical symptoms are caused by the eggs. As the leading cause of schistosomiasis in the world, it is the most prevalent parasite in humans. Unlike other flukes (trematodes) in which sexes are not separate (monoecious), schistosomes are unique in that adults are divided into males and females, thus, gonochoric. However, a permanent male-female pair, a condition called in copula, is required to become adults; for this, they are considered as hermaphrodites. The life cycle of schistosomes includes two hosts: humans as definitive hosts, where the parasite undergoes sexual reproduction, and snails as intermediate hosts, where a series of asexual reproduction takes place. S. mansoni is transmitted through water, where freshwater snails of the genus Biomphalaria act as intermediate hosts. The larvae are able to live in water and infect the hosts by directly penetrating the skin. Prevention of infection is done by improved sanitation and killing the snails. Infection is treated with praziquantel.
Schistosome eggs, which may become lodged within the hosts tissues, are the major cause of pathology in schistosomiasis. Some of the deposited eggs reach the outside environment by passing through the wall of the intestine; the rest are swept into the circulation and are filtered out in the periportal tracts of the liver, resulting in periportal fibrosis. Onset of egg laying in humans is sometimes associated with an onset of fever (Katayama fever). This “acute schistosomiasis” is not, however, as important as the chronic forms of the disease. For S. mansoni and S. japonicum, these are “intestinal” and “hepatic schistosomiasis”, associated with formation of granulomas around trapped eggs lodged in the intestinal wall or in the liver, respectively. The hepatic form of the disease is the most important, granulomas here giving rise to fibrosis of the liver and hepatosplenomegaly in severe cases. Symptoms and signs depend on the number and location of eggs trapped in the tissues. Initially, the inflammatory reaction is readily reversible. In the latter stages of the disease, the pathology is associated with collagen deposition and fibrosis, resulting in organ damage that may be only partially reversible.
Granuloma formation is initiated by antigens secreted by the miracidium through microscopic pores within the rigid egg shell, and the immune response to granuloma, rather than the direct action of egg antigens, causes the symptoms.[ The granulomas formed around the eggs impair blood flow in the liver and, as a consequence, induce portal hypertension. With time, collateral circulation is formed and the eggs disseminate into the lungs, where they cause more granulomas, pulmonary arteritis and, later, cor pulmonale. A contributory factor to portal hypertension is Symmers’ fibrosis, which develops around branches of the portal veins. This fibrosis occurs only many years after the infection and is presumed to be caused in part by soluble egg antigens and various immune cells that react to them. Recent research has shown that granuloma size is consistent with levels of IL-13, which plays a prominent role in granuloma formation and granuloma size. IL-13 receptor α 2 (IL-13Rα2) binds IL-13 with high affinity and blocks the effects of IL-13. Thus, this receptor is essential in preventing the progression of schistosomiasis from the acute to the chronic (and deadly) stage of disease. Synthetic IL-13Rα2 given to mice has resulted in significant decreases in granuloma size, implicating IL-13Rα2 as an important target in schistosomiasis. S. mansoni infection often occurs alongside those of viral hepatitis, either hepatitis B virus (HBV) or hepatitis C virus (HCV). This is due to high prevalence of schistosomiasis in areas where chronic viral hepatitis is prevalent. One important factor was the development of large reservoir of infection due to extensive schistosomiasis control programs that used intravenously administered tartar emetic since the 1960s. Co-infection is known to cause earlier liver deterioration and more severe illness.
Extracellular inorganic phosphate (ePi) has also been identified as a modulator of OPN expression.
- Fatherazi S, Matsa-Dunn D, Foster BL, Rutherford RB, Somerman MJ, Presland RB (January 2009). “Phosphate regulates osteopontin gene transcription”. Journal of Dental Research. 88 (1): 39–44. doi:10.1177/0022034508328072. PMC 3128439. PMID 19131315.
Stimulation of OPN expression also occurs upon exposure of cells to pro-inflammatory cytokines, classical mediators of acute inflammation (e.g. tumour necrosis factor α [TNFα], infterleukin-1β [IL-1β]), angiotensin II, transforming growth factor β (TGFβ) and parathyroid hormone (PTH), although a detailed mechanistic understanding of these regulatory pathways are not yet known. Hyperglycemia and hypoxia are also known to increase OPN expression.
- Guo H, Cai CQ, Schroeder RA, Kuo PC (January 2001). “Osteopontin is a negative feedback regulator of nitric oxide synthesis in murine macrophages”. Journal of Immunology. 166 (2): 1079–1086. doi:10.4049/jimmunol.166.2.1079. PMID 11145688.
- Ricardo SD, Franzoni DF, Roesener CD, Crisman JM, Diamond JR (May 2000). “Angiotensinogen and AT(1) antisense inhibition of osteopontin translation in rat proximal tubular cells”. American Journal of Physiology. Renal Physiology. 278 (5): F708–F716. doi:10.1152/ajprenal.2000.278.5.F708. PMID 10807582.
- Noda M, Rodan GA (February 1989). “Transcriptional regulation of osteopontin production in rat osteoblast-like cells by parathyroid hormone”. The Journal of Cell Biology. 108 (2): 713–718. doi:10.1083/jcb.108.2.713. PMC 2115413. PMID 2465299.
- Hullinger TG, Pan Q, Viswanathan HL, Somerman MJ (January 2001). “TGFbeta and BMP-2 activation of the OPN promoter: roles of smad- and hox-binding elements”. Experimental Cell Research. 262 (1): 69–74. doi:10.1006/excr.2000.5074. PMID 11120606.
- Sodhi CP, Phadke SA, Batlle D, Sahai A (April 2001). “Hypoxia and high glucose cause exaggerated mesangial cell growth and collagen synthesis: role of osteopontin”. American Journal of Physiology. Renal Physiology. 280 (4): F667–F674. doi:10.1152/ajprenal.2001.280.4.F667. PMID 11249858. S2CID 26145407.
Function
Apoptosis
OPN is an important anti-apoptotic factor in many circumstances. OPN blocks the activation-induced cell death of macrophages and T cells as well as fibroblasts and endothelial cells exposed to harmful stimuli. OPN prevents non-programmed cell death in inflammatory colitis.
- Denhardt DT, Noda M, O’Regan AW, Pavlin D, Berman JS (May 2001). “Osteopontin as a means to cope with environmental insults: regulation of inflammation, tissue remodeling, and cell survival”. The Journal of Clinical Investigation. 107 (9): 1055–1061. doi:10.1172/JCI12980. PMC 209291. PMID 11342566.
- Standal T, Borset M, Sundan A (September 2004). “Role of osteopontin in adhesion, migration, cell survival and bone remodeling”. Experimental Oncology. 26 (3): 179–184. PMID 15494684.
- Da Silva AP, Pollett A, Rittling SR, Denhardt DT, Sodek J, Zohar R (September 2006). “Exacerbated tissue destruction in DSS-induced acute colitis of OPN-null mice is associated with downregulation of TNF-alpha expression and non-programmed cell death”. Journal of Cellular Physiology. 208 (3): 629–639. doi:10.1002/jcp.20701. PMID 16741956. S2CID 33704508.
Biomineralization
OPN belongs to a family of secreted acidic proteins (SIBLINGs, Small Integrin Binding LIgand N-Glycosylated proteins) whose members have an abundance of negatively charged amino acids such as Asp and Glu. OPN also has a large number of consensus sequence sites for post-translational phosphorylation of Ser residues to form phosphoserine, providing additional negative charge. Contiguous stretches of high negative charge in OPN have been identified and named the polyAsp motif (poly-aspartic acid) and the ASARM motif (acidic serine- and aspartate-rich motif), with the latter sequence having multiple phosphorylation sites. This overall negative charge of OPN, along with its specific acidic motifs and the fact that OPN is an intrinsically disordered protein allowing for open and flexible structures, permit OPN to bind strongly to calcium atoms available at crystal surfaces in various biominerals. Such binding of OPN to various types of calcium-based biominerals ‒ such as calcium-phosphate mineral in bones and teeth, calcium-carbonate mineral in inner ear otoconia and avian eggshells, and calcium-oxalate mineral in kidney stones – acts as a mineralization inhibitor by stabilizing transient mineral precursor phases and by binding directly to crystal surfaces, all of which regulate crystal growth.
- Fisher LW, Fedarko NS (2003). “Six genes expressed in bones and teeth encode the current members of the SIBLING family of proteins”. Connective Tissue Research. 44 (Suppl 1): 33–40. doi:10.1080/03008200390152061. PMID 12952171. S2CID 29910722.
- Kalmar L, Homola D, Varga G, Tompa P (September 2012). “Structural disorder in proteins brings order to crystal growth in biomineralization”. Bone. 51 (3): 528–534. doi:10.1016/j.bone.2012.05.009. PMID 22634174.
- Christensen B, Nielsen MS, Haselmann KF, Petersen TE, Sørensen ES (August 2005). “Post-translationally modified residues of native human osteopontin are located in clusters: identification of 36 phosphorylation and five O-glycosylation sites and their biological implications”. The Biochemical Journal. 390 (Pt 1): 285–292. doi:10.1042/BJ20050341. PMC 1184582. PMID 15869464.
- David V, Martin A, Hedge AM, Drezner MK, Rowe PS (March 2011). “ASARM peptides: PHEX-dependent and -independent regulation of serum phosphate”. American Journal of Physiology. Renal Physiology. 300 (3): F783–F791. doi:10.1152/ajprenal.00304.2010. PMC 3064126. PMID 21177780.
- Martin A, David V, Laurence JS, Schwarz PM, Lafer EM, Hedge AM, Rowe PS (April 2008). “Degradation of MEPE, DMP1, and release of SIBLING ASARM-peptides (minhibins): ASARM-peptide(s) are directly responsible for defective mineralization in HYP”. Endocrinology. 149 (4): 1757–1772. doi:10.1210/en.2007-1205. PMC 2276704. PMID 18162525.
- Addison WN, Nakano Y, Loisel T, Crine P, McKee MD (October 2008). “MEPE-ASARM peptides control extracellular matrix mineralization by binding to hydroxyapatite: an inhibition regulated by PHEX cleavage of ASARM”. Journal of Bone and Mineral Research. 23 (10): 1638–1649. doi:10.1359/jbmr.080601. PMID 18597632. S2CID 7709872.
- Addison WN, Masica DL, Gray JJ, McKee MD (April 2010). “Phosphorylation-dependent inhibition of mineralization by osteopontin ASARM peptides is regulated by PHEX cleavage”. Journal of Bone and Mineral Research. 25 (4): 695–705. doi:10.1359/jbmr.090832. PMID 19775205. S2CID 25689595.
- Kurzbach D, Platzer G, Schwarz TC, Henen MA, Konrat R, Hinderberger D (August 2013). “Cooperative unfolding of compact conformations of the intrinsically disordered protein osteopontin”. Biochemistry. 52 (31): 5167–5175. doi:10.1021/bi400502c. PMC 3737600. PMID 23848319.
- Azzopardi PV, O’Young J, Lajoie G, Karttunen M, Goldberg HA, Hunter GK (February 2010). “Roles of electrostatics and conformation in protein-crystal interactions”. PLOS ONE. 5 (2): e9330. Bibcode:2010PLoSO…5.9330A. doi:10.1371/journal.pone.0009330. PMC 2824833. PMID 20174473.
- Hunter GK, O’Young J, Grohe B, Karttunen M, Goldberg HA (December 2010). “The flexible polyelectrolyte hypothesis of protein-biomineral interaction”. Langmuir. 26 (24): 18639–18646. doi:10.1021/la100401r. PMID 20527831.
- McKee MD, Nanci A (May 1995). “Postembedding colloidal-gold immunocytochemistry of noncollagenous extracellular matrix proteins in mineralized tissues”. Microscopy Research and Technique. 31 (1): 44–62. doi:10.1002/jemt.1070310105. PMID 7626799. S2CID 5767310.
- Takemura T, Sakagami M, Nakase T, Kubo T, Kitamura Y, Nomura S (September 1994). “Localization of osteopontin in the otoconial organs of adult rats”. Hearing Research. 79 (1–2): 99–104. doi:10.1016/0378-5955(94)90131-7. PMID 7806488. S2CID 4765287.
- Hincke MT, Nys Y, Gautron J, Mann K, Rodriguez-Navarro AB, McKee MD (January 2012). “The eggshell: structure, composition and mineralization”. Frontiers in Bioscience. 17 (4): 1266–1280. doi:10.2741/3985. PMID 22201802.
- McKee MD, Nanci A, Khan SR (December 1995). “Ultrastructural immunodetection of osteopontin and osteocalcin as major matrix components of renal calculi”. Journal of Bone and Mineral Research. 10 (12): 1913–1929. doi:10.1002/jbmr.5650101211. PMID 8619372. S2CID 37508279.
- O’Young J, Chirico S, Al Tarhuni N, Grohe B, Karttunen M, Goldberg HA, Hunter GK (2009). “Phosphorylation of osteopontin peptides mediates adsorption to and incorporation into calcium oxalate crystals”. Cells Tissues Organs. 189 (1–4): 51–55. doi:10.1159/000151724. PMID 18728346. S2CID 5534110.
- Chien YC, Masica DL, Gray JJ, Nguyen S, Vali H, McKee MD (August 2009). “Modulation of calcium oxalate dihydrate growth by selective crystal-face binding of phosphorylated osteopontin and polyaspartate peptide showing occlusion by sectoral (compositional) zoning”. The Journal of Biological Chemistry. 284 (35): 23491–23501. doi:10.1074/jbc.M109.021899. PMC 2749123. PMID 19581305.
- Sodek J, Ganss B, McKee MD (2000). “Osteopontin”. Critical Reviews in Oral Biology and Medicine. 11 (3): 279–303. doi:10.1177/10454411000110030101. PMID 11021631.
- Reznikov N, Hoac B, Buss DJ, Addison WN, Barros NM, McKee MD (September 2020). “Biological stenciling of mineralization in the skeleton: Local enzymatic removal of inhibitors in the extracellular matrix”. Bone. 138: 115447. doi:10.1016/j.bone.2020.115447. PMID 32454257. S2CID 218909350.
- McKee MD, Buss DJ, Reznikov N (March 2022). “Mineral tessellation in bone and the stenciling principle for extracellular matrix mineralization”. Journal of Structural Biology. 214 (1): 107823. doi:10.1016/j.jsb.2021.107823. PMID 34915130. S2CID 245187449.
OPN is a substrate protein for a number of enzymes whose actions may modulate the mineralization-inhibiting function of OPN. PHEX (phosphate-regulating endopeptidase homolog X-linked) is one such enzyme, which extensively degrades OPN, and whose inactivating gene mutations (in X-linked hypophosphatemia, XLH) lead to altered processing of OPN such that inhibitory OPN cannot be degraded and accumulates in the bone (and tooth) extracellular matrix, contributing locally to the osteomalacia (soft hypomineralized bones, and odontomalacia – soft teeth) characteristic of XLH. A relationship describing local, physiologic double-negative (inhibiting inhibitors) regulation of mineralization involving OPN has been termed the Stenciling Principle of mineralization, whereby enzyme-substrate pairs imprint mineralization patterns into the extracellular matrix (most notably described for bone) by degrading mineralization inhibitors (e.g. TNAP enzyme degrading pyrophosphate inhibition, and PHEX enzyme degrading osteopontin inhibition). In relation to mineralization diseases, the Stenciling Principle is particularly relevant to the osteomalacia and odontomalacia observed in hypophosphatasia and X-linked hypophosphatemia.
- McKee MD, Buss DJ, Reznikov N (March 2022). “Mineral tessellation in bone and the stenciling principle for extracellular matrix mineralization”. Journal of Structural Biology. 214 (1): 107823. doi:10.1016/j.jsb.2021.107823. PMID 34915130. S2CID 245187449.
- McKee MD, Hoac B, Addison WN, Barros NM, Millán JL, Chaussain C (October 2013). “Extracellular matrix mineralization in periodontal tissues: Noncollagenous matrix proteins, enzymes, and relationship to hypophosphatasia and X-linked hypophosphatemia”. Periodontology 2000. 63 (1): 102–122. doi:10.1111/prd.12029. PMC 3766584. PMID 23931057.
- Boukpessi T, Hoac B, Coyac BR, Leger T, Garcia C, Wicart P, et al. (February 2017). “Osteopontin and the dento-osseous pathobiology of X-linked hypophosphatemia”. Bone. 95: 151–161. doi:10.1016/j.bone.2016.11.019. PMID 27884786.
- Barros NM, Hoac B, Neves RL, Addison WN, Assis DM, Murshed M, et al. (March 2013). “Proteolytic processing of osteopontin by PHEX and accumulation of osteopontin fragments in Hyp mouse bone, the murine model of X-linked hypophosphatemia”. Journal of Bone and Mineral Research. 28 (3): 688–699. doi:10.1002/jbmr.1766. PMID 22991293. S2CID 20840491.
- Reznikov N, Hoac B, Buss DJ, Addison WN, Barros NM, McKee MD (September 2020). “Biological stenciling of mineralization in the skeleton: Local enzymatic removal of inhibitors in the extracellular matrix”. Bone. 138: 115447. doi:10.1016/j.bone.2020.115447. PMID 32454257. S2CID 218909350.
The Stenciling Principle describes a double-negative process (inhibition of inhibitors) that promotes mineralization in bone and other mineralized tissues, whereas the default condition of inhibition alone prevents mineralization elsewhere in soft connective tissues. The stenciling principle acts across multiple levels from the macroscale (skeleton/dentition vs soft connective tissues), to the microscale (for example, entheses, and the tooth attachment complex where the soft periodontal ligament is situated between mineralized tooth cementum and mineralized alveolar bone), and to the mesoscale (mineral tessellation). It relates to both small-molecule (e.g. pyrophosphate) and protein (e.g. osteopontin) inhibitors of mineralization, and promoters (enzymes, e.g. TNAP, PHEX) that degrade the inhibitors to permit and regulate mineralization. In this process, an organizational motif for bone mineral arises that we call crossfibrillar mineral tessellation where mineral formations – called tesselles – geometrically approximate prolate ellipsoids and traverse multiple collagen fibrils (laterally). Tesselle growth is directed by the structural anisotropy of collagen, being spatially restrained in the shorter transverse tesselle dimensions (averaging 1.6 × 0.8 × 0.8 μm, aspect ratio 2, length range 1.5–2.5 μm). Temporo-spatially, the tesselles abut in 3D (close ellipsoid packing) to fill the volume of lamellar bone extracellular matrix. Poorly mineralized interfacial gaps between adjacent tesselles remain discernable even in mature lamellar bone. Tessellation of a same, small basic unit to form larger structural assemblies results in numerous 3D interfaces, allows dissipation of critical stresses, and enables fail-safe cyclic deformations. Incomplete tessellation in osteomalacia/odontomalacia may explain why soft osteomalacic bones buckle and deform under loading.
- Marc D. McKee Mineral tessellation in bone and the stenciling principle for extracellular matrix mineralization Journal of Structural Biology Volume 214, Issue 1, March 2022, doi.org/10.1016/j.jsb.2021.107823
Along with its role in the regulation of normal mineralization within the extracellular matrices of bones and teeth, OPN is also upregulated at sites of pathologic, ectopic calcification – such as for example, in urolithiasis and vascular calcification ‒ presumably at least in part to inhibit debilitating mineralization in these soft tissues.
- McKee MD, Addison WN, Kaartinen MT (2005). “Hierarchies of extracellular matrix and mineral organization in bone of the craniofacial complex and skeleton”. Cells Tissues Organs. 181 (3–4): 176–188. doi:10.1159/000091379. PMID 16612083. S2CID 40705942.
- Steitz SA, Speer MY, McKee MD, Liaw L, Almeida M, Yang H, Giachelli CM (December 2002). “Osteopontin inhibits mineral deposition and promotes regression of ectopic calcification”. The American Journal of Pathology. 161 (6): 2035–2046. doi:10.1016/S0002-9440(10)64482-3. PMC 1850905. PMID 12466120.
- Giachelli CM (March 1999). “Ectopic calcification: gathering hard facts about soft tissue mineralization”. The American Journal of Pathology. 154 (3): 671–675. doi:10.1016/S0002-9440(10)65313-8. PMC 1866412. PMID 10079244.
- Kaartinen MT, Murshed M, Karsenty G, McKee MD (April 2007). “Osteopontin upregulation and polymerization by transglutaminase 2 in calcified arteries of Matrix Gla protein-deficient mice”. The Journal of Histochemistry and Cytochemistry. 55 (4): 375–386. doi:10.1369/jhc.6A7087.2006. PMID 17189522
- McKee MD, Nanci A, Khan SR (December 1995). “Ultrastructural immunodetection of osteopontin and osteocalcin as major matrix components of renal calculi”. Journal of Bone and Mineral Research. 10 (12): 1913–1929. doi:10.1002/jbmr.5650101211. PMID 8619372. S2CID 37508279.
Bone remodeling
Osteopontin has been implicated as an important factor in bone remodeling. Specifically, OPN anchors osteoclasts to the surface of bones where it is immobilized by its mineral-binding properties allowing subsequent usage of its RGD motif for osteoclast integrin binding for cell attachment and migration. OPN at bone surfaces is located in a thin organic layer, the so-called lamina limitans. The organic part of bone is about 20% of the dry weight, and counts in, other than osteopontin, collagen type I, osteocalcin, osteonectin, and alkaline phosphatase. Collagen type I counts for 90% of the protein mass. The inorganic part of bone is the mineral hydroxyapatite, Ca10(PO4)6(OH)2. Loss of bone may lead to osteoporosis, as the bone is depleted for calcium if this is not supplied in the diet.
- Choi ST, Kim JH, Kang EJ, Lee SW, Park MC, Park YB, Lee SK (December 2008). “Osteopontin might be involved in bone remodelling rather than in inflammation in ankylosing spondylitis”. Rheumatology. 47 (12): 1775–1779. doi:10.1093/rheumatology/ken385. PMID 18854347.
- McKee MD, Nanci A (1996). “Osteopontin: an interfacial extracellular matrix protein in mineralized tissues”. Connective Tissue Research. 35 (1–4): 197–205. doi:10.3109/03008209609029192. PMID 9084658.
- Reinholt FP, Hultenby K, Oldberg A, Heinegård D (June 1990). “Osteopontin–a possible anchor of osteoclasts to bone”. Proceedings of the National Academy of Sciences of the United States of America. 87 (12): 4473–4475. Bibcode:1990PNAS…87.4473R. doi:10.1073/pnas.87.12.4473. PMC 54137. PMID 1693772.
OPN serves to initiate the process by which osteoclasts develop their ruffled borders to begin bone resorption. OPN contains and RGD integrin-binding motif
Once activated, osteoclasts move to areas of microfracture in the bone by chemotaxis. Osteoclasts lie in small cavities called Howship’s lacunae, formed from the digestion of the underlying bone. The sealing zone is the attachment of the osteoclast’s plasma membrane to the underlying bone. Sealing zones are bounded by belts of specialized adhesion structures called podosomes. Attachment to the bone matrix is facilitated by integrin receptors, such as αvβ3, via the specific amino acid motif Arg-Gly-Asp in bone matrix proteins, such as osteopontin. The osteoclast releases hydrogen ions through the action of carbonic anhydrase (H2O + CO2 → HCO3− + H+) through the ruffled border into the resorptive cavity, acidifying and aiding dissolution of the mineralized bone matrix into Ca2+, H3PO4, H2CO3, water and other substances. Dysfunction of the carbonic anhydrase has been documented to cause some forms of osteopetrosis. Hydrogen ions are pumped against a high concentration gradient by proton pumps, specifically a unique vacuolar-ATPase. This enzyme has been targeted in the prevention of osteoporosis. In addition, several hydrolytic enzymes, such as members of the cathepsin and matrix metalloprotease (MMP) groups, are released to digest the organic components of the matrix. These enzymes are released into the compartment by lysosomes. Of these hydrolytic enzymes, cathepsin K is of most importance.
Cell activation
Activated T cells are promoted by IL-12 to differentiate towards the Th1 type, producing cytokines including IL-12 and IFNγ. OPN inhibits production of the Th2 cytokine IL-10, which leads to enhanced Th1 response. OPN influences cell-mediated immunity and has Th1 cytokine functions. It enhances B cell immunoglobulin production and proliferation. OPN also induces mast cell degranulation. IgE-mediated anaphylaxis is significantly reduced in OPN knock-out mice compared to wild-type mice. The role of OPN in activation of macrophages has also been implicated in a cancer since OPN-producing tumors were able to induce macrophage activation compared to OPN-deficient tumors.
- Wang KX, Denhardt DT (2008). “Osteopontin: role in immune regulation and stress responses”. Cytokine & Growth Factor Reviews. 19 (5–6): 333–345. doi:10.1016/j.cytogfr.2008.08.001. PMID 18952487.
- Nagasaka A, Matsue H, Matsushima H, Aoki R, Nakamura Y, Kambe N, et al. (February 2008). “Osteopontin is produced by mast cells and affects IgE-mediated degranulation and migration of mast cells”. European Journal of Immunology. 38 (2): 489–499. doi:10.1002/eji.200737057. PMID 18200503.
- Crawford HC, Matrisian LM, Liaw L (November 1998). “Distinct roles of osteopontin in host defense activity and tumor survival during squamous cell carcinoma progression in vivo”. Cancer Research. 58 (22): 5206–5215. PMID 9823334.
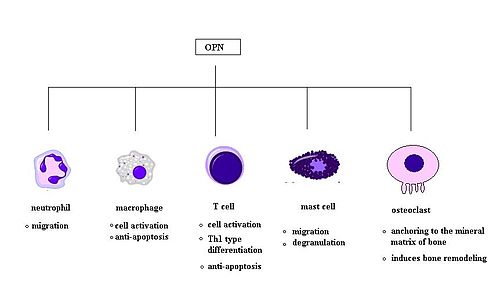
Chemotaxis
OPN plays an important role in neutrophil recruitment in alcoholic liver disease. OPN is important for the migration of neutrophil in vitro. In addition, OPN recruits inflammatory cells to arthritis joints in the collagen-induced arthritis model of rheumatoid arthritis. A recent in vitro study in 2008 has found that OPN plays a role in mast cell migration. Here OPN knock-out mast cells were cultured and they observed a decreased level of chemotaxis in these cells compared to wildtype mast cells. OPN was also found to act as a macrophage chemotactic factor. In rhesus monkey, OPN prevents macrophages from leaving the accumulation site in brains, indicating an increased level of chemotaxis.
- Banerjee A, Apte UM, Smith R, Ramaiah SK (March 2006). “Higher neutrophil infiltration mediated by osteopontin is a likely contributing factor to the increased susceptibility of females to alcoholic liver disease”. The Journal of Pathology. 208 (4): 473–485. doi:10.1002/path.1917. PMID 16440289. S2CID 45879742.
- Nagasaka A, Matsue H, Matsushima H, Aoki R, Nakamura Y, Kambe N, et al. (February 2008). “Osteopontin is produced by mast cells and affects IgE-mediated degranulation and migration of mast cells”. European Journal of Immunology. 38 (2): 489–499. doi:10.1002/eji.200737057. PMID 18200503.
- Apte UM, Banerjee A, McRee R, Wellberg E, Ramaiah SK (August 2005). “Role of osteopontin in hepatic neutrophil infiltration during alcoholic steatohepatitis”. Toxicology and Applied Pharmacology. 207 (1): 25–38. doi:10.1016/j.taap.2004.12.018. PMID 15885730.
- Koh A, da Silva AP, Bansal AK, Bansal M, Sun C, Lee H, et al. (December 2007). “Role of osteopontin in neutrophil function”. Immunology. 122 (4): 466–475. doi:10.1111/j.1365-2567.2007.02682.x. PMC 2266047. PMID 17680800.
- Ohshima S, Kobayashi H, Yamaguchi N, Nishioka K, Umeshita-Sasai M, Mima T, et al. (April 2002). “Expression of osteopontin at sites of bone erosion in a murine experimental arthritis model of collagen-induced arthritis: possible involvement of osteopontin in bone destruction in arthritis”. Arthritis and Rheumatism. 46 (4): 1094–1101. doi:10.1002/art.10143. PMID 11953989.
- Sakata M, Tsuruha JI, Masuko-Hongo K, Nakamura H, Matsui T, Sudo A, et al. (July 2001). “Autoantibodies to osteopontin in patients with osteoarthritis and rheumatoid arthritis”. The Journal of Rheumatology. 28 (7): 1492–1495. PMID 11469452.
- Burdo TH, Wood MR, Fox HS (June 2007). “Osteopontin prevents monocyte recirculation and apoptosis”. Journal of Leukocyte Biology. 81 (6): 1504–1511. doi:10.1189/jlb.1106711. PMC 2490714. PMID 17369493.
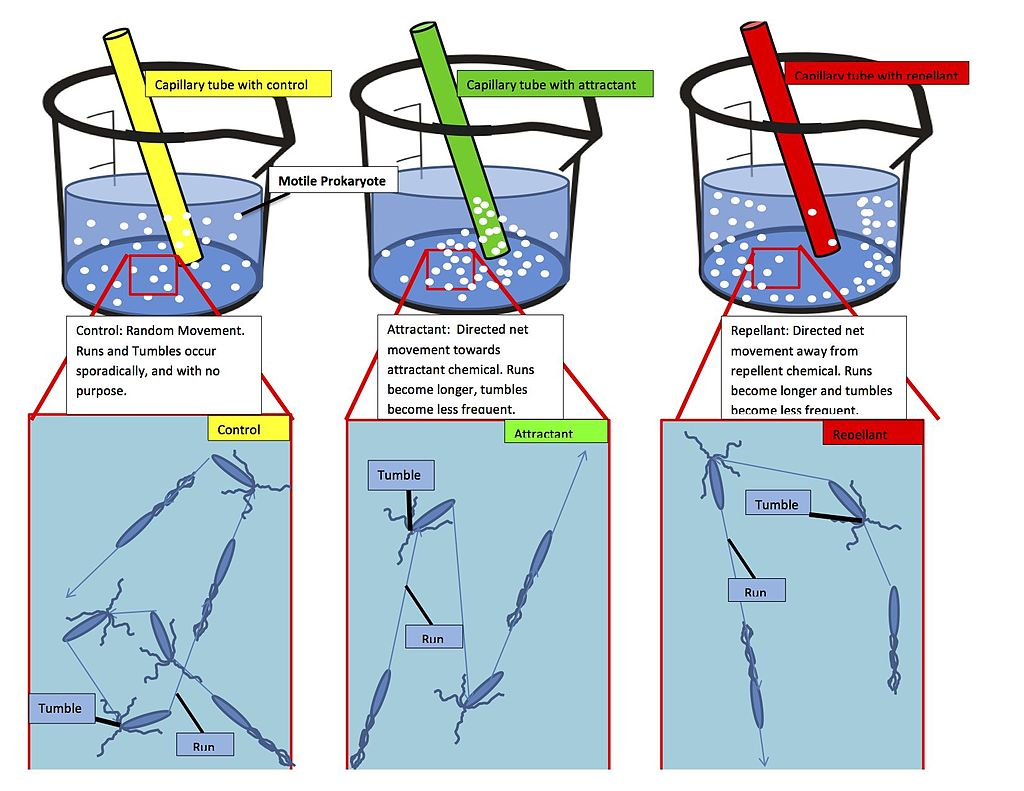
Chemotaxis (from chemo- + taxis) is the movement of an organism or entity in response to a chemical stimulus. Somatic cells, bacteria, and other single-cell or multicellular organisms direct their movements according to certain chemicals in their environment. This is important for bacteria to find food (e.g., glucose) by swimming toward the highest concentration of food molecules, or to flee from poisons (e.g., phenol). In multicellular organisms, chemotaxis is critical to early development (e.g., movement of sperm towards the egg during fertilization) and development (e.g., migration of neurons or lymphocytes) as well as in normal function and health (e.g., migration of leukocytes during injury or infection). In addition, it has been recognized that mechanisms that allow chemotaxis in animals can be subverted during cancer metastasis. The aberrant chemotaxis of leukocytes and lymphocytes also contribute to inflammatory diseases such as atherosclerosis, asthma, and arthritis. Sub-cellular components, such as the polarity patch generated by mating yeast, may also display chemotactic behavior. Positive chemotaxis occurs if the movement is toward a higher concentration of the chemical in question; negative chemotaxis if the movement is in the opposite direction. Chemically prompted kinesis (randomly directed or nondirectional) can be called chemokinesis.
- Chisholm, Hugh, ed. (1911). “Chemotaxis” . Encyclopædia Britannica. Vol. 6 (11th ed.). Cambridge University Press. p. 77.
- de Oliveira S, Rosowski EE, Huttenlocher A (May 2016). “Neutrophil migration in infection and wound repair: going forward in reverse”. Nature Reviews. Immunology. 16 (6): 378–91. doi:10.1038/nri.2016.49. PMC 5367630. PMID 27231052.
- Stuelten CH, Parent CA, Montell DJ (May 2018). “Cell motility in cancer invasion and metastasis: insights from simple model organisms”. Nature Reviews. Cancer. 18 (5): 296–312. doi:10.1038/nrc.2018.15. PMC 6790333. PMID 29546880.
- Li J, Ley K (January 2015). “Lymphocyte migration into atherosclerotic plaque”. Arteriosclerosis, Thrombosis, and Vascular Biology. 35 (1): 40–9. doi:10.1161/ATVBAHA.114.303227. PMC 4429868. PMID 25301842.
- Gelfand EW (October 2017). “Importance of the leukotriene B4-BLT1 and LTB4-BLT2 pathways in asthma”. Seminars in Immunology. 33: 44–51. doi:10.1016/j.smim.2017.08.005. PMC 5679233. PMID 29042028.
- Planagumà A, Domènech T, Pont M, Calama E, García-González V, López R, Aulí M, López M, Fonquerna S, Ramos I, de Alba J, Nueda A, Prats N, Segarra V, Miralpeix M, Lehner MD (October 2015). “Combined anti CXC receptors 1 and 2 therapy is a promising anti-inflammatory treatment for respiratory diseases by reducing neutrophil migration and activation”. Pulmonary Pharmacology & Therapeutics. 34: 37–45. doi:10.1016/j.pupt.2015.08.002. PMID 26271598.
- Rana AK, Li Y, Dang Q, Yang F (December 2018). “Monocytes in rheumatoid arthritis: Circulating precursors of macrophages and osteoclasts and, their heterogeneity and plasticity role in RA pathogenesis”. International Immunopharmacology. 65: 348–359. doi:10.1016/j.intimp.2018.10.016. PMID 30366278. S2CID 53116963.
- Ghose, Debraj; Jacobs, Katherine; Ramirez, Samuel; Elston, Timothy; Lew, Daniel (1 June 2021). “Chemotactic movement of a polarity site enables yeast cells to find their mates”. Proceedings of the National Academy of Sciences. 118 (22): e2025445118. Bibcode:2021PNAS..11825445G. doi:10.1073/pnas.2025445118. ISSN 0027-8424. PMC 8179161. PMID 34050026.
Immune system
OPN binds to several integrin receptors including α4β1, α9β1, and α9β4 expressed by leukocytes. These receptors have been well-established to function in cell adhesion, migration, and survival in these cells.
Osteopontin (OPN) is expressed in a range of immune cells, including macrophages, neutrophils, dendritic cells, microglia and T and B cells, with varying kinetics. OPN is reported to act as an immune modulator in a variety of manners Firstly, it has chemotactic properties, which promote cell recruitment to inflammatory sites. It also functions as an adhesion protein, involved in cell attachment and wound healing. In addition, OPN mediates cell activation and cytokine production, as well as promoting cell survival by regulating apoptosis. The following examples are found.
- Wang KX, Denhardt DT (2008). “Osteopontin: role in immune regulation and stress responses”. Cytokine & Growth Factor Reviews. 19 (5–6): 333–345. doi:10.1016/j.cytogfr.2008.08.001. PMID 18952487.
Cell adhesion molecules (CAMs) are a subset of cell surface proteins that are involved in the binding of cells with other cells or with the extracellular matrix (ECM), in a process called cell adhesion. In essence, CAMs help cells stick to each other and to their surroundings. CAMs are crucial components in maintaining tissue structure and function. In fully developed animals, these molecules play an integral role in generating force and movement and consequently ensuring that organs are able to execute their functions normally. In addition to serving as “molecular glue”, CAMs play important roles in the cellular mechanisms of growth, contact inhibition, and apoptosis. Aberrant expression of CAMs may result in a wide range of pathologies, ranging from frostbite to cancer.
There are four major superfamilies or groups of CAMs: the immunoglobulin super family of cell adhesion molecules (IgCAMs), Cadherins, Integrins, and the Superfamily of C-type of lectin-like domains proteins (CTLDs). Proteoglycans are also considered to be a class of CAMs. One classification system involves the distinction between calcium-independent CAMs and calcium-dependent CAMs. The Ig-superfamily CAMs do not depend on Ca2+ while integrins, cadherins and selectins depend on Ca2+. In addition, integrins participate in cell–matrix interactions, while other CAM families participate in cell–cell interactions. For the role of CAMs in the formation and stabilization of neural synapses, see Synaptic stabilization.
- Cell+Adhesion+Molecules at the U.S. National Library of Medicine Medical Subject Headings (MeSH)
- Chothia, C.; Jones, E. Y. (1997). “The molecular structure of cell adhesion molecules”. Annual Review of Biochemistry. 66: 823–862. doi:10.1146/annurev.biochem.66.1.823. ISSN 0066-4154. PMID 9242926.
- Gumbiner, B. M. (1996-02-09). “Cell adhesion: the molecular basis of tissue architecture and morphogenesis”. Cell. 84 (3): 345–357. doi:10.1016/s0092-8674(00)81279-9. ISSN 0092-8674. PMID 8608588.
- Korthuis RJ, Anderson DC, Granger DN (March 1994). “Role of neutrophil-endothelial cell adhesion in inflammatory disorders”. J Crit Care. 9 (1): 47–71. doi:10.1016/0883-9441(94)90032-9. ISSN 0883-9441. PMID 8199653.
- “Single-pass transmembrane adhesion and structural proteins”. membranome. College of Pharmacy, University of Michigan. Retrieved October 20, 2018.in Membranome database
- Chothia C, Jones EY (1997). “The molecular structure of cell adhesion molecules”. Annu. Rev. Biochem. 66: 823–62. doi:10.1146/annurev.biochem.66.1.823. PMID 9242926. S2CID 6298053.
- Brackenbury R, Rutishauser U, Edelman GM (January 1981). “Distinct calcium-independent and calcium-dependent adhesion systems of chicken embryo cells”. Proc. Natl. Acad. Sci. U.S.A. 78 (1): 387–91. Bibcode:1981PNAS…78..387B. doi:10.1073/pnas.78.1.387. PMC 319058. PMID 6165990.
- Lodish, Harvey; Berk, Arnold; Zipursky, S. Lawrence; Matsudaira, Paul; Baltimore, David; Darnell, James (2000-01-01). “Cell–Cell Adhesion and Communication”.
- Brown, K; Yamada, K (1995), “The Role of Integrins during Vertebrae Development”, Developmental Biology, 6 (2): 69–77, doi:10.1016/s1044-5781(06)80016-2
Distinct calcium-independent and calcium-dependent adhesion systems of chicken embryo cells.
Three criteria have been used to distinguish among different systems of embryonic cell adhesion: dependence on Ca2+, involvement of particular cell-surface molecules, and binding specificity. The characterization of the adhesion with respect to cell-surface molecules was carried out by using specific antibodies against the neural and liver cell adhesion molecules (N-CAM and L-CAM) and antibodies raised against retinal cells prepared by limited trypsinization in the presence of Ca2+ (called “T/Ca cells”). Aggregation of cells prepared from retina or brain without Ca2+ did not require Ca2+ and was inhibited by anti-(N-CAM) antibodies but not by anti-(L-CAM) or anti-T/Ca cell antibodies. In contrast, cells obtained from the same tissues in the presence of Ca2+ did require Ca2+ to aggregate. This aggregation was inhibited by anti-T/Ca cell antibodies but not by anti-(N-CAM) or anti-(L-CAM) antibodies. Hepatocyte aggregation also required Ca2+ and was inhibited only by anti-(L-CAM) antibodies. These results define three antigenically distinct cell adhesion systems in the embryo and raise the possibility that additional systems will be found. The neural Ca2+-independent system displayed a limited tissue specificity, mediating binding to neural but not liver cells. In contrast, the Ca2+-dependent systems of both neural and liver cells caused binding to all cell types tested. The Ca2+-dependent system was most active in retinal cells from 6-7 day embryos, whereas the Ca2+-independent system was most active at later times during development. In addition, treatments that inhibited the Ca2+-independent or Ca2+-dependent systems had very different effects on the fasciculation of neurites from dorsal root ganglia. All of the results suggest that Ca2+-independent and Ca2+-dependent adhesion systems play different functional roles during embryogenesis.
- Brackenbury R, Rutishauser U, Edelman GM. Distinct calcium-independent and calcium-dependent adhesion systems of chicken embryo cells. Proc Natl Acad Sci U S A. 1981 Jan;78(1):387-91. doi: 10.1073/pnas.78.1.387. PMID: 6165990; PMCID: PMC319058.
- Edelman GM. Surface modulation in cell recognition and cell growth. Science. 1976 Apr 16;192(4236):218–226. [PubMed] [Google Scholar]
- Thiery JP, Brackenbury R, Rutishauser U, Edelman GM. Adhesion among neural cells of the chick embryo. II. Purification and characterization of a cell adhesion molecule from neural retina. J Biol Chem. 1977 Oct 10;252(19):6841–6845. [PubMed] [Google Scholar]
- Hausman RE, Moscona AA. Isolation of retina-specific cell-aggregating factor from membranes of embryonic neural retina tissue. Proc Natl Acad Sci U S A. 1976 Oct;73(10):3594–3598. [PMC free article] [PubMed] [Google Scholar]
- Balsamo J, Lilien J. Functional identification of three components which mediate tissue-type specific embryonic cell adhesion. Nature. 1974 Oct 11;251(5475):522–524. [PubMed] [Google Scholar]
- Merrell R, Gottlieb DI, Glaser L. Embryonal cell surface recognition. Extraction of an active plasma membrane component. J Biol Chem. 1975 Jul 25;250(14):5655–5659. [PubMed] [Google Scholar]
- Jakoi ER, Marchase RB. Ligatin from embryonic chick neural retina. J Cell Biol. 1979 Mar;80(3):642–650. [PMC free article] [PubMed] [Google Scholar]
- Rutishauser U, Thiery JP, Brackenbury R, Sela BA, Edelman GM. Mechanisms of adhesion among cells from neural tissues of the chick embryo. Proc Natl Acad Sci U S A. 1976 Feb;73(2):577–581. [PMC free article] [PubMed] [Google Scholar]
- Brackenbury R, Thiery JP, Rutishauser U, Edelman GM. Adhesion among neural cells of the chick embryo. I. An immunological assay for molecules involved in cell-cell binding. J Biol Chem. 1977 Oct 10;252(19):6835–6840. [PubMed] [Google Scholar]
- Bertolotti R, Rutishauser U, Edelman GM. A cell surface molecule involved in aggregation of embryonic liver cells. Proc Natl Acad Sci U S A. 1980 Aug;77(8):4831–4835. [PMC free article] [PubMed] [Google Scholar]
- Steinberg MS, Armstrong PB, Granger RE. On the recovery of adhesiveness by trypsin-dissociated cells. J Membr Biol. 1973;13(2):97–128. [PubMed] [Google Scholar]
- Urushihara H, Ozaki HS, Takeichi M. Immunological detection of cell surface components related with aggregation of Chinese hamster and chick embryonic cells. Dev Biol. 1979 May;70(1):206–216. [PubMed] [Google Scholar]
- Takeichi M. Functional correlation between cell adhesive properties and some cell surface proteins. J Cell Biol. 1977 Nov;75(2 Pt 1):464–474. [PMC free article] [PubMed] [Google Scholar]
- Takeichi M, Ozaki HS, Tokunaga K, Okada TS. Experimental manipulation of cell surface to affect cellular recognition mechanisms. Dev Biol. 1979 May;70(1):195–205. [PubMed] [Google Scholar]
- Urushihara H, Takeichi M. Cell-cell adhesion molecule: identification of a glycoprotein relevant to the Ca2+-independent aggregation of Chinese hamster fibroblasts. Cell. 1980 Jun;20(2):363–371. [PubMed] [Google Scholar]
- Grunwald GB, Geller RL, Lilien J. Enzymatic dissection of embryonic cell adhesive mechanisms. J Cell Biol. 1980 Jun;85(3):766–776. [PMC free article] [PubMed] [Google Scholar]
- Coon HG. Clonal stability and phenotypic expression of chick cartilage cells in vitro. Proc Natl Acad Sci U S A. 1966 Jan;55(1):66–73. [PMC free article] [PubMed] [Google Scholar]
- Walther BT, Ohman R, Roseman S. A quantitative assay for intercellular adhesion. Proc Natl Acad Sci U S A. 1973 May;70(5):1569–1573. [PMC free article] [PubMed] [Google Scholar]
- Sela BA, Lis H, Sharon N, Sachs L. Isolectins from wax bean with differential agglutination of normal and transformed mammalian cells. Biochim Biophys Acta. 1973 May 17;310(1):273–277. [PubMed] [Google Scholar]
- Rutishauser U, Gall WE, Edelman GM. Adhesion among neural cells of the chick embryo. IV. Role of the cell surface molecule CAM in the formation of neurite bundles in cultures of spinal ganglia. J Cell Biol. 1978 Nov;79(2 Pt 1):382–393. [PMC free article] [PubMed] [Google Scholar]
- McGuire EJ. Intercellular adhesive selectivity. II. Properties of embryonic chick liver cell-cell adhesion. J Cell Biol. 1976 Jan;68(1):90–100. [PMC free article] [PubMed] [Google Scholar]
- Rutishauser U, Thiery JP, Brackenbury R, Edelman GM. Adhesion among neural cells of the chick embryo. III. Relationship of the surface molecule CAM to cell adhesion and the development of histotypic patterns. J Cell Biol. 1978 Nov;79(2 Pt 1):371–381. [PMC free article] [PubMed] [Google Scholar]
- Buskirk DR, Thiery JP, Rutishauser U, Edelman GM. Antibodies to a neural cell adhesion molecule disrupt histogenesis in cultured chick retinae. Nature. 1980 Jun 12;285(5765):488–489. [PubMed] [Google Scholar]
- Rutishauser U, Edelman GM. Effects of fasciculation on the outgrowth of neurites from spinal ganglia in culture. J Cell Biol. 1980 Nov;87(2 Pt 1):370–378. [PMC free article] [PubMed] [Google Scholar]
Clinical significance
The fact that OPN interacts with multiple cell surface receptors that are ubiquitously expressed makes it an active player in many physiological and pathological processes including wound healing, bone turnover, tumorigenesis, inflammation, ischemia, and immune responses. Manipulation of plasma (or local) OPN levels may be useful in the treatment of autoimmune diseases, cancer metastasis, bone (and tooth) mineralization diseases, osteoporosis, and some forms of stress.
- Wang KX, Denhardt DT (2008). “Osteopontin: role in immune regulation and stress responses”. Cytokine & Growth Factor Reviews. 19 (5–6): 333–345. doi:10.1016/j.cytogfr.2008.08.001. PMID 18952487.
Autoimmune diseases
OPN has been implicated in pathogenesis of rheumatoid arthritis. OPN-R, the thrombin-cleaved form of OPN, is elevated rheumatoid arthritis affected joints. However, the role of OPN in rheumatoid arthritis is still unclear. One group found that OPN knock-out mice were protected against arthritis. while others were not able to reproduce this observation.
- Yumoto K, Ishijima M, Rittling SR, Tsuji K, Tsuchiya Y, Kon S, et al. (April 2002). “Osteopontin deficiency protects joints against destruction in anti-type II collagen antibody-induced arthritis in mice”. Proceedings of the National Academy of Sciences of the United States of America. 99 (7): 4556–4561. Bibcode:2002PNAS…99.4556Y. doi:10.1073/pnas.052523599. PMC 123686. PMID 11930008.
- Jacobs JP, Pettit AR, Shinohara ML, Jansson M, Cantor H, Gravallese EM, et al. (August 2004). “Lack of requirement of osteopontin for inflammation, bone erosion, and cartilage damage in the K/BxN model of autoantibody-mediated arthritis”. Arthritis and Rheumatism. 50 (8): 2685–2694. doi:10.1002/art.20381. PMID 15334485.
OPN has been found to play a role in other autoimmune diseases including autoimmune hepatitis, allergic airway disease (WTF is this?), and multiple sclerosis.
- Chabas D, Baranzini SE, Mitchell D, Bernard CC, Rittling SR, Denhardt DT, et al. (November 2001). “The influence of the proinflammatory cytokine, osteopontin, on autoimmune demyelinating disease”. Science. 294 (5547): 1731–1735. Bibcode:2001Sci…294.1731C. doi:10.1126/science.1062960. PMID 11721059. S2CID 86208485.
Allergy and asthma
Osteopontin has recently been associated with allergic inflammation and asthma. Expression of Opn is significantly increased in lung epithelial and subepithelial cells of asthmatic patients in comparison to healthy subjects. Opn expression is also upregulated in lungs of mice with allergic airway inflammation. The secreted form of Opn (Opn-s) plays a proinflammatory role during allergen sensitization (OVA/Alum), as neutralization of Opn-s during that phase results in significantly milder allergic airway inflammation. In contrast, neutralization of Opn-s during antigenic challenge exacerbates allergic airway disease. These effects of Opn-s are mainly mediated by the regulation of Th2-suppressing plasmacytoid dendritic cells (DCs) during primary sensitization and Th2-promoting conventional DCs during secondary antigenic challenge. OPN deficiency was also reported to protect against remodeling and bronchial hyperresponsiveness (BHR), again using a chronic allergen-challenge model of airway remodeling. Furthermore, it was recently demonstrated that OPN expression is upregulated in human asthma, is associated with remodeling changes and its subepithelial expression correlates to disease severity. OPN has also been reported to be increased in the sputum supernatant of smoking asthmatics, as well as the BALF and bronchial tissue of smoking controls and asthmatics.
- Xanthou G, Alissafi T, Semitekolou M, Simoes DC, Economidou E, Gaga M, et al. (May 2007). “Osteopontin has a crucial role in allergic airway disease through regulation of dendritic cell subsets”. Nature Medicine. 13 (5): 570–578. doi:10.1038/nm1580. PMC 3384679. PMID 17435770.
- Simoes DC, Xanthou G, Petrochilou K, Panoutsakopoulou V, Roussos C, Gratziou C (May 2009). “Osteopontin deficiency protects against airway remodeling and hyperresponsiveness in chronic asthma”. American Journal of Respiratory and Critical Care Medicine. 179 (10): 894–902. doi:10.1164/rccm.200807-1081OC. PMID 19234104.
- Samitas K, Zervas E, Vittorakis S, Semitekolou M, Alissafi T, Bossios A, et al. (February 2011). “Osteopontin expression and relation to disease severity in human asthma”. The European Respiratory Journal. 37 (2): 331–341. doi:10.1183/09031936.00017810. PMID 20562127.
- Hillas G, Loukides S, Kostikas K, Simoes D, Petta V, Konstantellou E, et al. (January 2013). “Increased levels of osteopontin in sputum supernatant of smoking asthmatics”. Cytokine. 61 (1): 251–255. doi:10.1016/j.cyto.2012.10.002. PMID 23098767.
- Samitas K, Zervas E, Xanthou G, Panoutsakopoulou V, Gaga M (March 2013). “Osteopontin is increased in the bronchoalveolar lavage fluid and bronchial tissue of smoking asthmatics”. Cytokine. 61 (3): 713–715. doi:10.1016/j.cyto.2012.12.028. PMID 23384656.
Colitis
Opn is up-regulated in inflammatory bowel disease (IBD). Opn expression is highly up-regulated in intestinal immune and non-immune cells and in the plasma of patients with Crohn’s disease (CD) and ulcerative colitis (UC), as well as in the colon and plasma of mice with experimental colitis. Increased plasma Opn levels are related to the severity of CD inflammation, and certain Opn gene (Spp1) haplotypes are modifiers of CD susceptibility. Opn has also a proinflammatory role in TNBS- and dextran sulfate sodium (DSS)-induced colitis, which are mouse models for IBD. Opn was found highly expressed by a specific dendritic cell (DC) subset derived from murine mesenteric lymph nodes (MLNs) and is highly proinflammatory for colitis. Dendritic cells are important for the development of intestinal inflammation in humans with IBD and in mice with experimental colitis. Opn expression by this inflammatory MLN DC subset is crucial for their pathogenic action during colitis.
- Gassler N, Autschbach F, Gauer S, Bohn J, Sido B, Otto HF, et al. (November 2002). “Expression of osteopontin (Eta-1) in Crohn disease of the terminal ileum”. Scandinavian Journal of Gastroenterology. 37 (11): 1286–1295. doi:10.1080/003655202761020560. PMID 12465727. S2CID 6381267.
- Sato T, Nakai T, Tamura N, Okamoto S, Matsuoka K, Sakuraba A, et al. (September 2005). “Osteopontin/Eta-1 upregulated in Crohn’s disease regulates the Th1 immune response”. Gut. 54 (9): 1254–1262. doi:10.1136/gut.2004.048298. PMC 1774642. PMID 16099792.
- Mishima R, Takeshima F, Sawai T, Ohba K, Ohnita K, Isomoto H, et al. (February 2007). “High plasma osteopontin levels in patients with inflammatory bowel disease”. Journal of Clinical Gastroenterology. 41 (2): 167–172. doi:10.1097/MCG.0b013e31802d6268. PMID 17245215. S2CID 25039321.
- Kourepini E, Aggelakopoulou M, Alissafi T, Paschalidis N, Simoes DC, Panoutsakopoulou V (March 2014). “Osteopontin expression by CD103- dendritic cells drives intestinal inflammation”. Proceedings of the National Academy of Sciences of the United States of America. 111 (9): E856–E865. Bibcode:2014PNAS..111E.856K. doi:10.1073/pnas.1316447111. PMC 3948306. PMID 24550510.
Cancer
It has been shown that OPN drives IL-17 production; OPN is overexpressed in a variety of cancers, including lung cancer, breast cancer, colorectal cancer, stomach cancer, ovarian cancer, papillary thyroid carcinoma, melanoma and pleural mesothelioma; OPN contributes both glomerulonephritis and tubulointerstitial nephritis; and OPN is found in atheromatous plaques within arteries. Thus, manipulation of plasma OPN levels may be useful in the treatment of autoimmune diseases, cancer metastasis, osteoporosis and some forms of stress.
- Steinman L (February 2007). “A brief history of T(H)17, the first major revision in the T(H)1/T(H)2 hypothesis of T cell-mediated tissue damage”. Nature Medicine. 13 (2): 139–145. doi:10.1038/nm1551. PMID 17290272. S2CID 10640379.
- Wang KX, Denhardt DT (2008). “Osteopontin: role in immune regulation and stress responses”. Cytokine & Growth Factor Reviews. 19 (5–6): 333–345. doi:10.1016/j.cytogfr.2008.08.001. PMID 18952487.
Osteopontin is implicated in PDAC (pancreatic adenocarcinoma) disease progression. It is expressed as one of three splice variants in PDAC, with osteopontin-a expressed in nearly all PDAC, osteopontin-b expression correlating with survival, and osteopontin-c correlating with metastatic disease . Because PDAC secretes alternatively spliced forms of osteopontin, it shows potential for tumor- and disease stage-specific targeting. Although the exact mechanisms of osteopontin signaling in PDAC are unknown, it binds to CD44 and integrins to trigger processes such as tumor progression and complement inhibition. Osteopontin also drives metastasis by triggering the release of vascular endothelial growth factor (VEGF) and matrix metalloprotease (MMP), which is inhibited by knocking down osteopontin. This process is also stimulated by nicotine, which is the proposed mechanism by which smokers experience elevated PC risk. Osteopontin is being explored as a marker for PC. It was found to perform better than CA19.9 in discerning IPMN [80] and resectable PDAC from pancreatitis . Antiosteopontin antibodies are being developed, including hu1A12, which inhibited metastasis in an in vivo study and also when hybridized with the anti-VEGF antibody bevacizumab . At least one clinical trial is exploring the use of osteopontin as a marker of intratumoral hypoxia. However, this marker remains relatively unexplored.
Nicotine thing can’t be right…at least not in connection to smokers. The internet says French fries and ketchup have more nicotine, among other things. I’m going to guess smokers (and I don’t include vapers with smokers) have some protection as with all other organs.
- Clay R, Siddiqi SA (January 2019). “Recent advances in molecular diagnostics and therapeutic targets for pancreatic cancer”. Theranostic Approach for Pancreatic Cancer: 325–367. doi:10.1016/B978-0-12-819457-7.00016-5. ISBN 9780128194577. S2CID 214323538.
- Clay R, Siddiqi SA (2019-01-01). “Recent advances in molecular diagnostics and therapeutic targets for pancreatic cancer”. Theranostic Approach for Pancreatic Cancer: 325–367. doi:10.1016/B978-0-12-819457-7.00016-5. ISBN 9780128194577. S2CID 214323538.
Osteopontin is also implicated in excessive scar-formation and a gel has been developed to inhibit its effect.
- Mori R, Shaw TJ, Martin P (January 2008). “Molecular mechanisms linking wound inflammation and fibrosis: knockdown of osteopontin leads to rapid repair and reduced scarring”. The Journal of Experimental Medicine. 205 (1): 43–51. doi:10.1084/jem.20071412. PMC2234383. PMID18180311.
- “Gel ‘to speed up wound healing'”. BBC News. 22 January 2008.
AOM1, an anti-osteopontin monoclonal antibody drug developed by Pfizer, Inc. to inhibit osteopontin, showed promise at preventing progression of large metastatic tumors in mouse models of NSCLC.
- Shojaei F, Scott N, Kang X, Lappin PB, Fitzgerald AA, Karlicek S, et al. (March 2012). “Osteopontin induces growth of metastatic tumors in a preclinical model of non-small lung cancer”. Journal of Experimental & Clinical Cancer Research. 31 (1): 26. doi:10.1186/1756-9966-31-26. PMC 3325875. PMID 22444159.
- Farrokhi V, Chabot JR, Neubert H, Yang Z (May 2018). “Assessing the Feasibility of Neutralizing Osteopontin with Various Therapeutic Antibody Modalities”. Scientific Reports. 8 (1): 7781. Bibcode:2018NatSR…8.7781F. doi:10.1038/s41598-018-26187-w. PMC 5958109. PMID 29773891.
Even though Opn promotes metastasis and can be used as a cancer biomarker, latest studies described novel protecting functions of the molecule on innate cell populations during tumor development. Particularly, maintenance of a pool of natural killer (NK) cells with optimal immune function is crucial for host defense against cancerous tumor formation. A study in PNAS describes iOpn is an essential molecular component responsible for maintenance of functional NK cell expansion. Absence of iOPN results in failure to maintain normal NK cellularity and increased cell death following stimulation by cytokine IL-15. OPN-deficient NK cells fail to successfully navigate the contraction phase of the immune response, resulting in impaired expansion of long-lived NK cells and defective responses to tumor cells. In addition, plasmacytoid dendritic cells (pDCs) protect from melanoma, and this effect is mediated by type I IFNs. A study in JCB showed that a specific fragment (SLAYGLR) of the Opn protein can render pDCs more “fit” to protect from melanoma development. This was achieved by activation of a novel α4 integrin/IFN-β axis which is MyD88-independent and operates via a PI3K/mTOR/IRF3 pathway.
- Leavenworth JW, Verbinnen B, Wang Q, Shen E, Cantor H. Intracellular osteopontin regulates homeostasis and function of natural killer cells. Proc Natl Acad Sci U S A. 2015 Jan 13;112(2):494-9. doi: 10.1073/pnas.1423011112. Epub 2014 Dec 30. PMID: 25550515; PMCID: PMC4299239.
- Drobits B, Holcmann M, Amberg N, Swiecki M, Grundtner R, Hammer M, Colonna M, Sibilia M. Imiquimod clears tumors in mice independent of adaptive immunity by converting pDCs into tumor-killing effector cells. J Clin Invest. 2012 Feb;122(2):575-85. doi: 10.1172/JCI61034. Epub 2012 Jan 17. PMID: 22251703; PMCID: PMC3266798.
- Davina Camargo Madeira Simoes, Nikolaos Paschalidis, Evangelia Kourepini, Vily Panoutsakopoulou; An integrin axis induces IFN-β production in plasmacytoid dendritic cells. J Cell Biol 5 September 2022; 221 (9): e202102055. doi: https://doi.org/10.1083/jcb.202102055
Heart failure
Osteopontin is minimally expressed under normal conditions, but accumulates quickly as heart function declines. Specifically, it plays a central role in the remodeling response to myocardial infarction, and is dramatically upregulated in hypertrophic (HCM) and dilated cardiomyopathy (DCM). Once abundant, it stimulates a wide range of physiological changes in the myocardium, including angiogenesis, local production of cytokines, differentiation of myofibroblasts, increased deposition of extracellular matrix, and hypertrophy of cardiomyocytes. Taken together, these processes remodel the structure of the heart, in effect reducing its ability to function normally, and increasing risk for heart failure.
- Singh M, Dalal S, Singh K (November 2014). “Osteopontin: At the cross-roads of myocyte survival and myocardial function”. Life Sciences. 118 (1): 1–6. doi:10.1016/j.lfs.2014.09.014. PMC 4254317. PMID 25265596.
- Singh M, Foster CR, Dalal S, Singh K (March 2010). “Osteopontin: role in extracellular matrix deposition and myocardial remodeling post-MI”. Journal of Molecular and Cellular Cardiology. 48 (3): 538–543. doi:10.1016/j.yjmcc.2009.06.015. PMC 2823840. PMID 19573532.
- Shirakawa K, Sano M (July 2021). “Osteopontin in Cardiovascular Diseases”. Biomolecules. 11 (7): 1047. doi:10.3390/biom11071047. PMC 8301767. PMID 34356671.
- Graf K, Do YS, Ashizawa N, Meehan WP, Giachelli CM, Marboe CC, et al. (November 1997). “Myocardial osteopontin expression is associated with left ventricular hypertrophy”. Circulation. 96 (9): 3063–3071. doi:10.1161/01.CIR.96.9.3063. PMID 9386176.
Parkinson’s disease
OPN plays a role in oxidative and nitrosative stress, apoptosis, mitochondrial dysfunction, and excitotoxicity, which are also involved in the pathogenesis of Parkinson’s disease. PD patients serum and cerebrospinal fluid (CSF) concentrations of OPN were studied, it shows that OPN levels in the body fluid is elevated in PD patients.
- Maetzler W, Berg D, Schalamberidze N, Melms A, Schott K, Mueller JC, et al. (March 2007). “Osteopontin is elevated in Parkinson’s disease and its absence leads to reduced neurodegeneration in the MPTP model”. Neurobiology of Disease. 25 (3): 473–482. doi:10.1016/j.nbd.2006.10.020. PMID 17188882. S2CID 30275400.
Muscle disease and injury
Evidence is accumulating that suggests that osteopontin plays a number of roles in diseases of skeletal muscle, such as Duchenne muscular dystrophy. Osteopontin has been described as a component of the inflammatory environment of dystrophic and injured muscles, and has also been shown to increase scarring of diaphragm muscles of aged dystrophic mice. A recent study has identified osteopontin as a determinant of disease severity in patients with Duchenne muscular dystrophy. This study found that a mutation in the osteopontin gene promoter, known to cause low levels of osteopontin expression, is associated with a decrease in age to loss of ambulation and muscle strength in patients with Duchenne muscular dystrophy.
- Uaesoontrachoon K, Yoo HJ, Tudor EM, Pike RN, Mackie EJ, Pagel CN (April 2008). “Osteopontin and skeletal muscle myoblasts: association with muscle regeneration and regulation of myoblast function in vitro”. The International Journal of Biochemistry & Cell Biology. 40 (10): 2303–2314. doi:10.1016/j.biocel.2008.03.020. PMID 18490187.
- Porter JD, Khanna S, Kaminski HJ, Rao JS, Merriam AP, Richmonds CR, et al. (February 2002). “A chronic inflammatory response dominates the skeletal muscle molecular signature in dystrophin-deficient mdx mice”. Human Molecular Genetics. 11 (3): 263–272. doi:10.1093/hmg/11.3.263. PMID 11823445.
- Haslett JN, Sanoudou D, Kho AT, Bennett RR, Greenberg SA, Kohane IS, et al. (November 2002). “Gene expression comparison of biopsies from Duchenne muscular dystrophy (DMD) and normal skeletal muscle”. Proceedings of the National Academy of Sciences of the United States of America. 99 (23): 15000–15005. Bibcode:2002PNAS…9915000H. doi:10.1073/pnas.192571199. PMC 137534. PMID 12415109.
- Hirata A, Masuda S, Tamura T, Kai K, Ojima K, Fukase A, et al. (July 2003). “Expression profiling of cytokines and related genes in regenerating skeletal muscle after cardiotoxin injection: a role for osteopontin”. The American Journal of Pathology. 163 (1): 203–215. doi:10.1016/S0002-9440(10)63644-9. PMC 1868192. PMID 12819025.
- Vetrone SA, Montecino-Rodriguez E, Kudryashova E, Kramerova I, Hoffman EP, Liu SD, et al. (June 2009). “Osteopontin promotes fibrosis in dystrophic mouse muscle by modulating immune cell subsets and intramuscular TGF-beta”. The Journal of Clinical Investigation. 119 (6): 1583–1594. doi:10.1172/JCI37662. PMC 2689112. PMID 19451692.
- Pegoraro E, Hoffman EP, Piva L, Gavassini BF, Cagnin S, Ermani M, et al. (January 2011). “SPP1 genotype is a determinant of disease severity in Duchenne muscular dystrophy”. Neurology. 76 (3): 219–226. doi:10.1212/WNL.0b013e318207afeb. PMC 3034396. PMID 21178099.
Hip osteoarthritis
An increase in Plasma OPN levels has been observed in patients with idiopathic hip OA. Furthermore, a correlation between OPN plasma levels and the severity of the disease has been noted.
- El Deeb S, Abdelnaby R, Khachab A, Bläsius K, Tingart M, Rath B (July 2016). “Osteopontin as a biochemical marker and severity indicator for idiopathic hip osteoarthritis”. Hip International. 26 (4): 397–403. doi:10.5301/hipint.5000361. PMID 27229171. S2CID 40819265.
Fertilized egg implantation
OPN is expressed in endometrial cells during implantation. Due to the production of progesterone by the ovaries, OPN is up-regulated immensely to aid in this process. The endometrium must undergo decidualization, the process in which the endometrium undergoes changes to prepare for implantation, which will lead to the attachment of the embryo. The endometrium houses stromal cells that will differentiate to produce an optimal environment for the embryo to attach (decidualization). OPN is a vital protein for stromal cell proliferation and differentiation as well as it binds to the receptor αvβ3 to assist with adhesion. OPN along with decidualization ultimately encourages the successful implantation of the early embryo. An OPN gene knock-out results in attachment instability at the maternal-fetal interface.
- Kang YJ, Forbes K, Carver J, Aplin JD (April 2014). “The role of the osteopontin-integrin αvβ3 interaction at implantation: functional analysis using three different in vitro models”. Human Reproduction. 29 (4): 739–749. doi:10.1093/humrep/det433. PMID 24442579.
- Johnson GA, Burghardt RC, Bazer FW, Spencer TE (November 2003). “Osteopontin: roles in implantation and placentation”. Biology of Reproduction. 69 (5): 1458–1471. doi:10.1095/biolreprod.103.020651. PMID 12890718.
References
- GRCh38: Ensembl release 89: ENSG00000118785 – Ensembl, May 2017
- GRCm38: Ensembl release 89: ENSMUSG00000029304 – Ensembl, May 2017
- “Human PubMed Reference:”. National Center for Biotechnology Information, U.S. National Library of Medicine.
- “Mouse PubMed Reference:”. National Center for Biotechnology Information, U.S. National Library of Medicine.
- “Entrez Gene: SPP1 secreted phosphoprotein 1”.
- Kalmar L, Homola D, Varga G, Tompa P (September 2012). “Structural disorder in proteins brings order to crystal growth in biomineralization”. Bone. 51 (3): 528–534. doi:10.1016/j.bone.2012.05.009. PMID 22634174.
- Wang KX, Denhardt DT (2008). “Osteopontin: role in immune regulation and stress responses”. Cytokine & Growth Factor Reviews. 19 (5–6): 333–345. doi:10.1016/j.cytogfr.2008.08.001. PMID 18952487.
- Rangaswami H, Bulbule A, Kundu GC (February 2006). “Osteopontin: role in cell signaling and cancer progression”. Trends in Cell Biology. 16 (2): 79–87. doi:10.1016/j.tcb.2005.12.005. PMID 16406521.
- Young MF, Kerr JM, Termine JD, Wewer UM, Wang MG, McBride OW, Fisher LW (August 1990). “cDNA cloning, mRNA distribution and heterogeneity, chromosomal location, and RFLP analysis of human osteopontin (OPN)”. Genomics. 7 (4): 491–502. doi:10.1016/0888-7543(90)90191-V. PMID 1974876.
- Kiefer MC, Bauer DM, Barr PJ (April 1989). “The cDNA and derived amino acid sequence for human osteopontin”. Nucleic Acids Research. 17 (8): 3306. doi:10.1093/nar/17.8.3306. PMC 317745. PMID 2726470.
- Crosby AH, Edwards SJ, Murray JC, Dixon MJ (May 1995). “Genomic organization of the human osteopontin gene: exclusion of the locus from a causative role in the pathogenesis of dentinogenesis imperfecta type II”. Genomics. 27 (1): 155–160. doi:10.1006/geno.1995.1018. PMID 7665163.
- Barros NM, Hoac B, Neves RL, Addison WN, Assis DM, Murshed M, et al. (March 2013). “Proteolytic processing of osteopontin by PHEX and accumulation of osteopontin fragments in Hyp mouse bone, the murine model of X-linked hypophosphatemia”. Journal of Bone and Mineral Research. 28 (3): 688–699. doi:10.1002/jbmr.1766. PMID 22991293. S2CID 20840491.
- Laffón A, García-Vicuña R, Humbría A, Postigo AA, Corbí AL, de Landázuri MO, Sánchez-Madrid F (August 1991). “Upregulated expression and function of VLA-4 fibronectin receptors on human activated T cells in rheumatoid arthritis”. The Journal of Clinical Investigation. 88 (2): 546–552. doi:10.1172/JCI115338. PMC 295383. PMID 1830891.
- Seiffge D (December 1996). “Protective effects of monoclonal antibody to VLA-4 on leukocyte adhesion and course of disease in adjuvant arthritis in rats”. The Journal of Rheumatology. 23 (12): 2086–2091. PMID 8970045.
- Reinholt FP, Hultenby K, Oldberg A, Heinegård D (June 1990). “Osteopontin–a possible anchor of osteoclasts to bone”. Proceedings of the National Academy of Sciences of the United States of America. 87 (12): 4473–4475. Bibcode:1990PNAS…87.4473R. doi:10.1073/pnas.87.12.4473. PMC 54137. PMID 1693772.
- Banerjee A, Apte UM, Smith R, Ramaiah SK (March 2006). “Higher neutrophil infiltration mediated by osteopontin is a likely contributing factor to the increased susceptibility of females to alcoholic liver disease”. The Journal of Pathology. 208 (4): 473–485. doi:10.1002/path.1917. PMID 16440289. S2CID 45879742.
- Sodek J, Batista Da Silva AP, Zohar R (May 2006). “Osteopontin and mucosal protection”. Journal of Dental Research. 85 (5): 404–415. doi:10.1177/154405910608500503. PMID 16632752. S2CID 25898774.[permanent dead link]
- Zohar R, Suzuki N, Suzuki K, Arora P, Glogauer M, McCulloch CA, Sodek J (July 2000). “Intracellular osteopontin is an integral component of the CD44-ERM complex involved in cell migration”. Journal of Cellular Physiology. 184 (1): 118–130. doi:10.1002/(SICI)1097-4652(200007)184:1<118::AID-JCP13>3.0.CO;2-Y. PMID 10825241. S2CID 11548419.
- Suzuki K, Zhu B, Rittling SR, Denhardt DT, Goldberg HA, McCulloch CA, Sodek J (August 2002). “Colocalization of intracellular osteopontin with CD44 is associated with migration, cell fusion, and resorption in osteoclasts”. Journal of Bone and Mineral Research. 17 (8): 1486–1497. doi:10.1359/jbmr.2002.17.8.1486. PMID 12162503. S2CID 21307088.
- Zhu B, Suzuki K, Goldberg HA, Rittling SR, Denhardt DT, McCulloch CA, Sodek J (January 2004). “Osteopontin modulates CD44-dependent chemotaxis of peritoneal macrophages through G-protein-coupled receptors: evidence of a role for an intracellular form of osteopontin”. Journal of Cellular Physiology. 198 (1): 155–167. doi:10.1002/jcp.10394. PMID 14584055. S2CID 21075107.
- Junaid A, Moon MC, Harding GE, Zahradka P (February 2007). “Osteopontin localizes to the nucleus of 293 cells and associates with polo-like kinase-1”. American Journal of Physiology. Cell Physiology. 292 (2): C919–C926. doi:10.1152/ajpcell.00477.2006. PMID 17005603.
- Shinohara ML, Kim HJ, Kim JH, Garcia VA, Cantor H (May 2008). “Alternative translation of osteopontin generates intracellular and secreted isoforms that mediate distinct biological activities in dendritic cells”. Proceedings of the National Academy of Sciences of the United States of America. 105 (20): 7235–7239. Bibcode:2008PNAS..105.7235S. doi:10.1073/pnas.0802301105. PMC 2438233. PMID 18480255.
- He B, Mirza M, Weber GF (April 2006). “An osteopontin splice variant induces anchorage independence in human breast cancer cells”. Oncogene. 25 (15): 2192–2202. doi:10.1038/sj.onc.1209248. PMID 16288209. S2CID 6632395.
- Mirza M, Shaughnessy E, Hurley JK, Vanpatten KA, Pestano GA, He B, Weber GF (February 2008). “Osteopontin-c is a selective marker of breast cancer”. International Journal of Cancer. 122 (4): 889–897. doi:10.1002/ijc.23204. PMID 17960616. S2CID 22146646.
- Ashizawa N, Graf K, Do YS, Nunohiro T, Giachelli CM, Meehan WP, et al. (November 1996). “Osteopontin is produced by rat cardiac fibroblasts and mediates A(II)-induced DNA synthesis and collagen gel contraction”. The Journal of Clinical Investigation. 98 (10): 2218–2227. doi:10.1172/JCI119031. PMC 507670. PMID 8941637.
- Murry CE, Giachelli CM, Schwartz SM, Vracko R (December 1994). “Macrophages express osteopontin during repair of myocardial necrosis”. The American Journal of Pathology. 145 (6): 1450–1462. PMC 1887495. PMID 7992848.
- Ikeda T, Shirasawa T, Esaki Y, Yoshiki S, Hirokawa K (December 1993). “Osteopontin mRNA is expressed by smooth muscle-derived foam cells in human atherosclerotic lesions of the aorta”. The Journal of Clinical Investigation. 92 (6): 2814–2820. doi:10.1172/JCI116901. PMC 288482. PMID 8254036.
- Uaesoontrachoon K, Yoo HJ, Tudor EM, Pike RN, Mackie EJ, Pagel CN (April 2008). “Osteopontin and skeletal muscle myoblasts: association with muscle regeneration and regulation of myoblast function in vitro”. The International Journal of Biochemistry & Cell Biology. 40 (10): 2303–2314. doi:10.1016/j.biocel.2008.03.020. PMID 18490187.
- Merry K, Dodds R, Littlewood A, Gowen M (April 1993). “Expression of osteopontin mRNA by osteoclasts and osteoblasts in modelling adult human bone”. Journal of Cell Science. 104 (4): 1013–1020. doi:10.1242/jcs.104.4.1013. PMID 8314886.
- Nakashima K, Zhou X, Kunkel G, Zhang Z, Deng JM, Behringer RR, de Crombrugghe B (January 2002). “The novel zinc finger-containing transcription factor osterix is required for osteoblast differentiation and bone formation”. Cell. 108 (1): 17–29. doi:10.1016/S0092-8674(01)00622-5. PMID 11792318. S2CID 14030684.
- Ducy P, Zhang R, Geoffroy V, Ridall AL, Karsenty G (May 1997). “Osf2/Cbfa1: a transcriptional activator of osteoblast differentiation”. Cell. 89 (5): 747–754. doi:10.1016/S0092-8674(00)80257-3. PMID 9182762. S2CID 16979723.
- Yucha C, Guthrie D (December 2003). “Renal homeostasis of calcium”. Nephrology Nursing Journal. 30 (6): 755–764. PMID 14730782.
- Prince CW, Butler WT (September 1987). “1,25-Dihydroxyvitamin D3 regulates the biosynthesis of osteopontin, a bone-derived cell attachment protein, in clonal osteoblast-like osteosarcoma cells”. Collagen and Related Research. 7 (4): 305–313. doi:10.1016/s0174-173x(87)80036-5. PMID 3478171.
- Oldberg A, Jirskog-Hed B, Axelsson S, Heinegård D (December 1989). “Regulation of bone sialoprotein mRNA by steroid hormones”. The Journal of Cell Biology. 109 (6 Pt 1): 3183–3186. doi:10.1083/jcb.109.6.3183. PMC 2115918. PMID 2592421.
- Chang PL, Prince CW (April 1991). “1 alpha,25-dihydroxyvitamin D3 stimulates synthesis and secretion of nonphosphorylated osteopontin (secreted phosphoprotein 1) in mouse JB6 epidermal cells”. Cancer Research. 51 (8): 2144–2150. PMID 2009532.
- Pereira TA, Vaz de Melo Trindade G, Trindade Santos E, Pereira FE, Souza MM (May 2021). “Praziquantel pharmacotherapy reduces systemic osteopontin levels and liver collagen content in murine schistosomiasis mansoni”. International Journal for Parasitology. 51 (6): 437–440. doi:10.1016/j.ijpara.2020.11.002. PMID 33493521. S2CID 231711719.
- “Editorial”. International Journal for Parasitology. 26 (8–9): 797–798. 1996-08-09. doi:10.1016/0020-7519(96)00065-3. ISSN 0020-7519.
- Fatherazi S, Matsa-Dunn D, Foster BL, Rutherford RB, Somerman MJ, Presland RB (January 2009). “Phosphate regulates osteopontin gene transcription”. Journal of Dental Research. 88 (1): 39–44. doi:10.1177/0022034508328072. PMC 3128439. PMID 19131315.
- Guo H, Cai CQ, Schroeder RA, Kuo PC (January 2001). “Osteopontin is a negative feedback regulator of nitric oxide synthesis in murine macrophages”. Journal of Immunology. 166 (2): 1079–1086. doi:10.4049/jimmunol.166.2.1079. PMID 11145688.
- Ricardo SD, Franzoni DF, Roesener CD, Crisman JM, Diamond JR (May 2000). “Angiotensinogen and AT(1) antisense inhibition of osteopontin translation in rat proximal tubular cells”. American Journal of Physiology. Renal Physiology. 278 (5): F708–F716. doi:10.1152/ajprenal.2000.278.5.F708. PMID 10807582.
- Noda M, Rodan GA (February 1989). “Transcriptional regulation of osteopontin production in rat osteoblast-like cells by parathyroid hormone”. The Journal of Cell Biology. 108 (2): 713–718. doi:10.1083/jcb.108.2.713. PMC 2115413. PMID 2465299.
- Hullinger TG, Pan Q, Viswanathan HL, Somerman MJ (January 2001). “TGFbeta and BMP-2 activation of the OPN promoter: roles of smad- and hox-binding elements”. Experimental Cell Research. 262 (1): 69–74. doi:10.1006/excr.2000.5074. PMID 11120606.
- Sodhi CP, Phadke SA, Batlle D, Sahai A (April 2001). “Hypoxia and high glucose cause exaggerated mesangial cell growth and collagen synthesis: role of osteopontin”. American Journal of Physiology. Renal Physiology. 280 (4): F667–F674. doi:10.1152/ajprenal.2001.280.4.F667. PMID 11249858. S2CID 26145407.
- Denhardt DT, Noda M, O’Regan AW, Pavlin D, Berman JS (May 2001). “Osteopontin as a means to cope with environmental insults: regulation of inflammation, tissue remodeling, and cell survival”. The Journal of Clinical Investigation. 107 (9): 1055–1061. doi:10.1172/JCI12980. PMC 209291. PMID 11342566.
- Standal T, Borset M, Sundan A (September 2004). “Role of osteopontin in adhesion, migration, cell survival and bone remodeling”. Experimental Oncology. 26 (3): 179–184. PMID 15494684.
- Da Silva AP, Pollett A, Rittling SR, Denhardt DT, Sodek J, Zohar R (September 2006). “Exacerbated tissue destruction in DSS-induced acute colitis of OPN-null mice is associated with downregulation of TNF-alpha expression and non-programmed cell death”. Journal of Cellular Physiology. 208 (3): 629–639. doi:10.1002/jcp.20701. PMID 16741956. S2CID 33704508.
- Fisher LW, Fedarko NS (2003). “Six genes expressed in bones and teeth encode the current members of the SIBLING family of proteins”. Connective Tissue Research. 44 (Suppl 1): 33–40. doi:10.1080/03008200390152061. PMID 12952171. S2CID 29910722.
- Christensen B, Nielsen MS, Haselmann KF, Petersen TE, Sørensen ES (August 2005). “Post-translationally modified residues of native human osteopontin are located in clusters: identification of 36 phosphorylation and five O-glycosylation sites and their biological implications”. The Biochemical Journal. 390 (Pt 1): 285–292. doi:10.1042/BJ20050341. PMC 1184582. PMID 15869464.
- David V, Martin A, Hedge AM, Drezner MK, Rowe PS (March 2011). “ASARM peptides: PHEX-dependent and -independent regulation of serum phosphate”. American Journal of Physiology. Renal Physiology. 300 (3): F783–F791. doi:10.1152/ajprenal.00304.2010. PMC 3064126. PMID 21177780.
- Martin A, David V, Laurence JS, Schwarz PM, Lafer EM, Hedge AM, Rowe PS (April 2008). “Degradation of MEPE, DMP1, and release of SIBLING ASARM-peptides (minhibins): ASARM-peptide(s) are directly responsible for defective mineralization in HYP”. Endocrinology. 149 (4): 1757–1772. doi:10.1210/en.2007-1205. PMC 2276704. PMID 18162525.
- Addison WN, Nakano Y, Loisel T, Crine P, McKee MD (October 2008). “MEPE-ASARM peptides control extracellular matrix mineralization by binding to hydroxyapatite: an inhibition regulated by PHEX cleavage of ASARM”. Journal of Bone and Mineral Research. 23 (10): 1638–1649. doi:10.1359/jbmr.080601. PMID 18597632. S2CID 7709872.
- Addison WN, Masica DL, Gray JJ, McKee MD (April 2010). “Phosphorylation-dependent inhibition of mineralization by osteopontin ASARM peptides is regulated by PHEX cleavage”. Journal of Bone and Mineral Research. 25 (4): 695–705. doi:10.1359/jbmr.090832. PMID 19775205. S2CID 25689595.
- Kurzbach D, Platzer G, Schwarz TC, Henen MA, Konrat R, Hinderberger D (August 2013). “Cooperative unfolding of compact conformations of the intrinsically disordered protein osteopontin”. Biochemistry. 52 (31): 5167–5175. doi:10.1021/bi400502c. PMC 3737600. PMID 23848319.
- Azzopardi PV, O’Young J, Lajoie G, Karttunen M, Goldberg HA, Hunter GK (February 2010). “Roles of electrostatics and conformation in protein-crystal interactions”. PLOS ONE. 5 (2): e9330. Bibcode:2010PLoSO…5.9330A. doi:10.1371/journal.pone.0009330. PMC 2824833. PMID 20174473.
- Hunter GK, O’Young J, Grohe B, Karttunen M, Goldberg HA (December 2010). “The flexible polyelectrolyte hypothesis of protein-biomineral interaction”. Langmuir. 26 (24): 18639–18646. doi:10.1021/la100401r. PMID 20527831.
- McKee MD, Nanci A (May 1995). “Postembedding colloidal-gold immunocytochemistry of noncollagenous extracellular matrix proteins in mineralized tissues”. Microscopy Research and Technique. 31 (1): 44–62. doi:10.1002/jemt.1070310105. PMID 7626799. S2CID 5767310.
- Takemura T, Sakagami M, Nakase T, Kubo T, Kitamura Y, Nomura S (September 1994). “Localization of osteopontin in the otoconial organs of adult rats”. Hearing Research. 79 (1–2): 99–104. doi:10.1016/0378-5955(94)90131-7. PMID 7806488. S2CID 4765287.
- Hincke MT, Nys Y, Gautron J, Mann K, Rodriguez-Navarro AB, McKee MD (January 2012). “The eggshell: structure, composition and mineralization”. Frontiers in Bioscience. 17 (4): 1266–1280. doi:10.2741/3985. PMID 22201802.
- McKee MD, Nanci A, Khan SR (December 1995). “Ultrastructural immunodetection of osteopontin and osteocalcin as major matrix components of renal calculi”. Journal of Bone and Mineral Research. 10 (12): 1913–1929. doi:10.1002/jbmr.5650101211. PMID 8619372. S2CID 37508279.
- O’Young J, Chirico S, Al Tarhuni N, Grohe B, Karttunen M, Goldberg HA, Hunter GK (2009). “Phosphorylation of osteopontin peptides mediates adsorption to and incorporation into calcium oxalate crystals”. Cells Tissues Organs. 189 (1–4): 51–55. doi:10.1159/000151724. PMID 18728346. S2CID 5534110.
- Chien YC, Masica DL, Gray JJ, Nguyen S, Vali H, McKee MD (August 2009). “Modulation of calcium oxalate dihydrate growth by selective crystal-face binding of phosphorylated osteopontin and polyaspartate peptide showing occlusion by sectoral (compositional) zoning”. The Journal of Biological Chemistry. 284 (35): 23491–23501. doi:10.1074/jbc.M109.021899. PMC 2749123. PMID 19581305.
- Sodek J, Ganss B, McKee MD (2000). “Osteopontin”. Critical Reviews in Oral Biology and Medicine. 11 (3): 279–303. doi:10.1177/10454411000110030101. PMID 11021631.
- Reznikov N, Hoac B, Buss DJ, Addison WN, Barros NM, McKee MD (September 2020). “Biological stenciling of mineralization in the skeleton: Local enzymatic removal of inhibitors in the extracellular matrix”. Bone. 138: 115447. doi:10.1016/j.bone.2020.115447. PMID 32454257. S2CID 218909350.
- McKee MD, Buss DJ, Reznikov N (March 2022). “Mineral tessellation in bone and the stenciling principle for extracellular matrix mineralization”. Journal of Structural Biology. 214 (1): 107823. doi:10.1016/j.jsb.2021.107823. PMID 34915130. S2CID 245187449.
- McKee MD, Hoac B, Addison WN, Barros NM, Millán JL, Chaussain C (October 2013). “Extracellular matrix mineralization in periodontal tissues: Noncollagenous matrix proteins, enzymes, and relationship to hypophosphatasia and X-linked hypophosphatemia”. Periodontology 2000. 63 (1): 102–122. doi:10.1111/prd.12029. PMC 3766584. PMID 23931057.
- Boukpessi T, Hoac B, Coyac BR, Leger T, Garcia C, Wicart P, et al. (February 2017). “Osteopontin and the dento-osseous pathobiology of X-linked hypophosphatemia”. Bone. 95: 151–161. doi:10.1016/j.bone.2016.11.019. PMID 27884786.
- Barros NM, Hoac B, Neves RL, Addison WN, Assis DM, Murshed M, et al. (March 2013). “Proteolytic processing of osteopontin by PHEX and accumulation of osteopontin fragments in Hyp mouse bone, the murine model of X-linked hypophosphatemia”. Journal of Bone and Mineral Research. 28 (3): 688–699. doi:10.1002/jbmr.1766. PMID 22991293. S2CID 20840491.
- Reznikov N, Hoac B, Buss DJ, Addison WN, Barros NM, McKee MD (September 2020). “Biological stenciling of mineralization in the skeleton: Local enzymatic removal of inhibitors in the extracellular matrix”. Bone. 138: 115447. doi:10.1016/j.bone.2020.115447. PMID 32454257. S2CID 218909350.
- McKee MD, Addison WN, Kaartinen MT (2005). “Hierarchies of extracellular matrix and mineral organization in bone of the craniofacial complex and skeleton”. Cells Tissues Organs. 181 (3–4): 176–188. doi:10.1159/000091379. PMID 16612083. S2CID 40705942.
- Steitz SA, Speer MY, McKee MD, Liaw L, Almeida M, Yang H, Giachelli CM (December 2002). “Osteopontin inhibits mineral deposition and promotes regression of ectopic calcification”. The American Journal of Pathology. 161 (6): 2035–2046. doi:10.1016/S0002-9440(10)64482-3. PMC 1850905. PMID 12466120.
- Giachelli CM (March 1999). “Ectopic calcification: gathering hard facts about soft tissue mineralization”. The American Journal of Pathology. 154 (3): 671–675. doi:10.1016/S0002-9440(10)65313-8. PMC 1866412. PMID 10079244.
- Kaartinen MT, Murshed M, Karsenty G, McKee MD (April 2007). “Osteopontin upregulation and polymerization by transglutaminase 2 in calcified arteries of Matrix Gla protein-deficient mice”. The Journal of Histochemistry and Cytochemistry. 55 (4): 375–386. doi:10.1369/jhc.6A7087.2006. PMID 17189522.
- Choi ST, Kim JH, Kang EJ, Lee SW, Park MC, Park YB, Lee SK (December 2008). “Osteopontin might be involved in bone remodelling rather than in inflammation in ankylosing spondylitis”. Rheumatology. 47 (12): 1775–1779. doi:10.1093/rheumatology/ken385. PMID 18854347.
- McKee MD, Nanci A (1996). “Osteopontin: an interfacial extracellular matrix protein in mineralized tissues”. Connective Tissue Research. 35 (1–4): 197–205. doi:10.3109/03008209609029192. PMID 9084658.
- Nagasaka A, Matsue H, Matsushima H, Aoki R, Nakamura Y, Kambe N, et al. (February 2008). “Osteopontin is produced by mast cells and affects IgE-mediated degranulation and migration of mast cells”. European Journal of Immunology. 38 (2): 489–499. doi:10.1002/eji.200737057. PMID 18200503.
- Crawford HC, Matrisian LM, Liaw L (November 1998). “Distinct roles of osteopontin in host defense activity and tumor survival during squamous cell carcinoma progression in vivo”. Cancer Research. 58 (22): 5206–5215. PMID 9823334.
- Apte UM, Banerjee A, McRee R, Wellberg E, Ramaiah SK (August 2005). “Role of osteopontin in hepatic neutrophil infiltration during alcoholic steatohepatitis”. Toxicology and Applied Pharmacology. 207 (1): 25–38. doi:10.1016/j.taap.2004.12.018. PMID 15885730.
- Koh A, da Silva AP, Bansal AK, Bansal M, Sun C, Lee H, et al. (December 2007). “Role of osteopontin in neutrophil function”. Immunology. 122 (4): 466–475. doi:10.1111/j.1365-2567.2007.02682.x. PMC 2266047. PMID 17680800.
- Ohshima S, Kobayashi H, Yamaguchi N, Nishioka K, Umeshita-Sasai M, Mima T, et al. (April 2002). “Expression of osteopontin at sites of bone erosion in a murine experimental arthritis model of collagen-induced arthritis: possible involvement of osteopontin in bone destruction in arthritis”. Arthritis and Rheumatism. 46 (4): 1094–1101. doi:10.1002/art.10143. PMID 11953989.
- Sakata M, Tsuruha JI, Masuko-Hongo K, Nakamura H, Matsui T, Sudo A, et al. (July 2001). “Autoantibodies to osteopontin in patients with osteoarthritis and rheumatoid arthritis”. The Journal of Rheumatology. 28 (7): 1492–1495. PMID 11469452.
- Burdo TH, Wood MR, Fox HS (June 2007). “Osteopontin prevents monocyte recirculation and apoptosis”. Journal of Leukocyte Biology. 81 (6): 1504–1511. doi:10.1189/jlb.1106711. PMC 2490714. PMID 17369493.
- Yumoto K, Ishijima M, Rittling SR, Tsuji K, Tsuchiya Y, Kon S, et al. (April 2002). “Osteopontin deficiency protects joints against destruction in anti-type II collagen antibody-induced arthritis in mice”. Proceedings of the National Academy of Sciences of the United States of America. 99 (7): 4556–4561. Bibcode:2002PNAS…99.4556Y. doi:10.1073/pnas.052523599. PMC 123686. PMID 11930008.
- Jacobs JP, Pettit AR, Shinohara ML, Jansson M, Cantor H, Gravallese EM, et al. (August 2004). “Lack of requirement of osteopontin for inflammation, bone erosion, and cartilage damage in the K/BxN model of autoantibody-mediated arthritis”. Arthritis and Rheumatism. 50 (8): 2685–2694. doi:10.1002/art.20381. PMID 15334485.
- Chabas D, Baranzini SE, Mitchell D, Bernard CC, Rittling SR, Denhardt DT, et al. (November 2001). “The influence of the proinflammatory cytokine, osteopontin, on autoimmune demyelinating disease”. Science. 294 (5547): 1731–1735. Bibcode:2001Sci…294.1731C. doi:10.1126/science.1062960. PMID 11721059. S2CID 86208485.
- Xanthou G, Alissafi T, Semitekolou M, Simoes DC, Economidou E, Gaga M, et al. (May 2007). “Osteopontin has a crucial role in allergic airway disease through regulation of dendritic cell subsets”. Nature Medicine. 13 (5): 570–578. doi:10.1038/nm1580. PMC 3384679. PMID 17435770.
- Simoes DC, Xanthou G, Petrochilou K, Panoutsakopoulou V, Roussos C, Gratziou C (May 2009). “Osteopontin deficiency protects against airway remodeling and hyperresponsiveness in chronic asthma”. American Journal of Respiratory and Critical Care Medicine. 179 (10): 894–902. doi:10.1164/rccm.200807-1081OC. PMID 19234104.
- Samitas K, Zervas E, Vittorakis S, Semitekolou M, Alissafi T, Bossios A, et al. (February 2011). “Osteopontin expression and relation to disease severity in human asthma”. The European Respiratory Journal. 37 (2): 331–341. doi:10.1183/09031936.00017810. PMID 20562127.
- Hillas G, Loukides S, Kostikas K, Simoes D, Petta V, Konstantellou E, et al. (January 2013). “Increased levels of osteopontin in sputum supernatant of smoking asthmatics”. Cytokine. 61 (1): 251–255. doi:10.1016/j.cyto.2012.10.002. PMID 23098767.
- Samitas K, Zervas E, Xanthou G, Panoutsakopoulou V, Gaga M (March 2013). “Osteopontin is increased in the bronchoalveolar lavage fluid and bronchial tissue of smoking asthmatics”. Cytokine. 61 (3): 713–715. doi:10.1016/j.cyto.2012.12.028. PMID 23384656.
- Gassler N, Autschbach F, Gauer S, Bohn J, Sido B, Otto HF, et al. (November 2002). “Expression of osteopontin (Eta-1) in Crohn disease of the terminal ileum”. Scandinavian Journal of Gastroenterology. 37 (11): 1286–1295. doi:10.1080/003655202761020560. PMID 12465727. S2CID 6381267.
- Sato T, Nakai T, Tamura N, Okamoto S, Matsuoka K, Sakuraba A, et al. (September 2005). “Osteopontin/Eta-1 upregulated in Crohn’s disease regulates the Th1 immune response”. Gut. 54 (9): 1254–1262. doi:10.1136/gut.2004.048298. PMC 1774642. PMID 16099792.
- Mishima R, Takeshima F, Sawai T, Ohba K, Ohnita K, Isomoto H, et al. (February 2007). “High plasma osteopontin levels in patients with inflammatory bowel disease”. Journal of Clinical Gastroenterology. 41 (2): 167–172. doi:10.1097/MCG.0b013e31802d6268. PMID 17245215. S2CID 25039321.
- Kourepini E, Aggelakopoulou M, Alissafi T, Paschalidis N, Simoes DC, Panoutsakopoulou V (March 2014). “Osteopontin expression by CD103- dendritic cells drives intestinal inflammation”. Proceedings of the National Academy of Sciences of the United States of America. 111 (9): E856–E865. Bibcode:2014PNAS..111E.856K. doi:10.1073/pnas.1316447111. PMC 3948306. PMID 24550510.
- Steinman L (February 2007). “A brief history of T(H)17, the first major revision in the T(H)1/T(H)2 hypothesis of T cell-mediated tissue damage”. Nature Medicine. 13 (2): 139–145. doi:10.1038/nm1551. PMID 17290272. S2CID 10640379.
- Clay R, Siddiqi SA (January 2019). “Recent advances in molecular diagnostics and therapeutic targets for pancreatic cancer”. Theranostic Approach for Pancreatic Cancer: 325–367. doi:10.1016/B978-0-12-819457-7.00016-5. ISBN 9780128194577. S2CID 214323538.
- Clay R, Siddiqi SA (2019-01-01). “Recent advances in molecular diagnostics and therapeutic targets for pancreatic cancer”. Theranostic Approach for Pancreatic Cancer: 325–367. doi:10.1016/B978-0-12-819457-7.00016-5. ISBN 9780128194577. S2CID 214323538.
- Mori R, Shaw TJ, Martin P (January 2008). “Molecular mechanisms linking wound inflammation and fibrosis: knockdown of osteopontin leads to rapid repair and reduced scarring”. The Journal of Experimental Medicine. 205 (1): 43–51. doi:10.1084/jem.20071412. PMC2234383. PMID18180311.
- “Gel ‘to speed up wound healing'”. BBC News. 22 January 2008.
- Shojaei F, Scott N, Kang X, Lappin PB, Fitzgerald AA, Karlicek S, et al. (March 2012). “Osteopontin induces growth of metastatic tumors in a preclinical model of non-small lung cancer”. Journal of Experimental & Clinical Cancer Research. 31 (1): 26. doi:10.1186/1756-9966-31-26. PMC 3325875. PMID 22444159.
- Farrokhi V, Chabot JR, Neubert H, Yang Z (May 2018). “Assessing the Feasibility of Neutralizing Osteopontin with Various Therapeutic Antibody Modalities”. Scientific Reports. 8 (1): 7781. Bibcode:2018NatSR…8.7781F. doi:10.1038/s41598-018-26187-w. PMC 5958109. PMID 29773891.
- Leavenworth JW, Verbinnen B, Wang Q, Shen E, Cantor H. Intracellular osteopontin regulates homeostasis and function of natural killer cells. Proc Natl Acad Sci U S A. 2015 Jan 13;112(2):494-9. doi: 10.1073/pnas.1423011112. Epub 2014 Dec 30. PMID: 25550515; PMCID: PMC4299239.
- Drobits B, Holcmann M, Amberg N, Swiecki M, Grundtner R, Hammer M, Colonna M, Sibilia M. Imiquimod clears tumors in mice independent of adaptive immunity by converting pDCs into tumor-killing effector cells. J Clin Invest. 2012 Feb;122(2):575-85. doi: 10.1172/JCI61034. Epub 2012 Jan 17. PMID: 22251703; PMCID: PMC3266798.
- Davina Camargo Madeira Simoes, Nikolaos Paschalidis, Evangelia Kourepini, Vily Panoutsakopoulou; An integrin axis induces IFN-β production in plasmacytoid dendritic cells. J Cell Biol 5 September 2022; 221 (9): e202102055. doi: https://doi.org/10.1083/jcb.202102055
- Singh M, Dalal S, Singh K (November 2014). “Osteopontin: At the cross-roads of myocyte survival and myocardial function”. Life Sciences. 118 (1): 1–6. doi:10.1016/j.lfs.2014.09.014. PMC 4254317. PMID 25265596.
- Singh M, Foster CR, Dalal S, Singh K (March 2010). “Osteopontin: role in extracellular matrix deposition and myocardial remodeling post-MI”. Journal of Molecular and Cellular Cardiology. 48 (3): 538–543. doi:10.1016/j.yjmcc.2009.06.015. PMC 2823840. PMID 19573532.
- Shirakawa K, Sano M (July 2021). “Osteopontin in Cardiovascular Diseases”. Biomolecules. 11 (7): 1047. doi:10.3390/biom11071047. PMC 8301767. PMID 34356671.
- Graf K, Do YS, Ashizawa N, Meehan WP, Giachelli CM, Marboe CC, et al. (November 1997). “Myocardial osteopontin expression is associated with left ventricular hypertrophy”. Circulation. 96 (9): 3063–3071. doi:10.1161/01.CIR.96.9.3063. PMID 9386176.
- Maetzler W, Berg D, Schalamberidze N, Melms A, Schott K, Mueller JC, et al. (March 2007). “Osteopontin is elevated in Parkinson’s disease and its absence leads to reduced neurodegeneration in the MPTP model”. Neurobiology of Disease. 25 (3): 473–482. doi:10.1016/j.nbd.2006.10.020. PMID 17188882. S2CID 30275400.
- Porter JD, Khanna S, Kaminski HJ, Rao JS, Merriam AP, Richmonds CR, et al. (February 2002). “A chronic inflammatory response dominates the skeletal muscle molecular signature in dystrophin-deficient mdx mice”. Human Molecular Genetics. 11 (3): 263–272. doi:10.1093/hmg/11.3.263. PMID 11823445.
- Haslett JN, Sanoudou D, Kho AT, Bennett RR, Greenberg SA, Kohane IS, et al. (November 2002). “Gene expression comparison of biopsies from Duchenne muscular dystrophy (DMD) and normal skeletal muscle”. Proceedings of the National Academy of Sciences of the United States of America. 99 (23): 15000–15005. Bibcode:2002PNAS…9915000H. doi:10.1073/pnas.192571199. PMC 137534. PMID 12415109.
- Hirata A, Masuda S, Tamura T, Kai K, Ojima K, Fukase A, et al. (July 2003). “Expression profiling of cytokines and related genes in regenerating skeletal muscle after cardiotoxin injection: a role for osteopontin”. The American Journal of Pathology. 163 (1): 203–215. doi:10.1016/S0002-9440(10)63644-9. PMC 1868192. PMID 12819025.
- Vetrone SA, Montecino-Rodriguez E, Kudryashova E, Kramerova I, Hoffman EP, Liu SD, et al. (June 2009). “Osteopontin promotes fibrosis in dystrophic mouse muscle by modulating immune cell subsets and intramuscular TGF-beta”. The Journal of Clinical Investigation. 119 (6): 1583–1594. doi:10.1172/JCI37662. PMC 2689112. PMID 19451692.
- Pegoraro E, Hoffman EP, Piva L, Gavassini BF, Cagnin S, Ermani M, et al. (January 2011). “SPP1 genotype is a determinant of disease severity in Duchenne muscular dystrophy”. Neurology. 76 (3): 219–226. doi:10.1212/WNL.0b013e318207afeb. PMC 3034396. PMID 21178099.
- El Deeb S, Abdelnaby R, Khachab A, Bläsius K, Tingart M, Rath B (July 2016). “Osteopontin as a biochemical marker and severity indicator for idiopathic hip osteoarthritis”. Hip International. 26 (4): 397–403. doi:10.5301/hipint.5000361. PMID 27229171. S2CID 40819265.
- Kang YJ, Forbes K, Carver J, Aplin JD (April 2014). “The role of the osteopontin-integrin αvβ3 interaction at implantation: functional analysis using three different in vitro models”. Human Reproduction. 29 (4): 739–749. doi:10.1093/humrep/det433. PMID 24442579.
- Johnson GA, Burghardt RC, Bazer FW, Spencer TE (November 2003). “Osteopontin: roles in implantation and placentation”. Biology of Reproduction. 69 (5): 1458–1471. doi:10.1095/biolreprod.103.020651. PMID 12890718.
Further reading
- Fujisawa R (March 2002). “[Recent advances in research on bone matrix proteins]”. Nihon Rinsho. Japanese Journal of Clinical Medicine. 60. 60 (Suppl 3): 72–78. PMID 11979972.
- Denhardt DT, Mistretta D, Chambers AF, Krishna S, Porter JF, Raghuram S, Rittling SR (2003). “Transcriptional regulation of osteopontin and the metastatic phenotype: evidence for a Ras-activated enhancer in the human OPN promoter”. Clinical & Experimental Metastasis. 20 (1): 77–84. doi:10.1023/A:1022550721404. PMID 12650610. S2CID 20286402.
- Yeatman TJ, Chambers AF (2003). “Osteopontin and colon cancer progression”. Clinical & Experimental Metastasis. 20 (1): 85–90. doi:10.1023/A:1022502805474. PMID 12650611. S2CID 25253392.
- O’Regan A (December 2003). “The role of osteopontin in lung disease”. Cytokine & Growth Factor Reviews. 14 (6): 479–488. doi:10.1016/S1359-6101(03)00055-8. PMID 14563350.
- Wai PY, Kuo PC (October 2004). “The role of Osteopontin in tumor metastasis”. The Journal of Surgical Research. 121 (2): 228–241. doi:10.1016/j.jss.2004.03.028. PMID 15501463.
- Konno S, Hizawa N, Nishimura M, Huang SK (December 2006). “Osteopontin: a potential biomarker for successful bee venom immunotherapy and a potential molecule for inhibiting IgE-mediated allergic responses”. Allergology International. 55 (4): 355–359. doi:10.2332/allergolint.55.355. PMID 17130676.
- Rodrigues LR, Teixeira JA, Schmitt FL, Paulsson M, Lindmark-Mänsson H (June 2007). “The role of osteopontin in tumor progression and metastasis in breast cancer”. Cancer Epidemiology, Biomarkers & Prevention. 16 (6): 1087–1097. doi:10.1158/1055-9965.EPI-06-1008. hdl:1822/7274. PMID 17548669.
- Ramaiah SK, Rittling S (August 2007). “Role of osteopontin in regulating hepatic inflammatory responses and toxic liver injury”. Expert Opinion on Drug Metabolism & Toxicology. 3 (4): 519–526. doi:10.1517/17425225.3.4.519. PMID 17696803.
External links
- Osteopontin at the U.S. National Library of Medicine Medical Subject Headings (MeSH)
- Overview of all the structural information available in the PDB for UniProt: P10451 (Osteopontin) at the PDBe-KB.