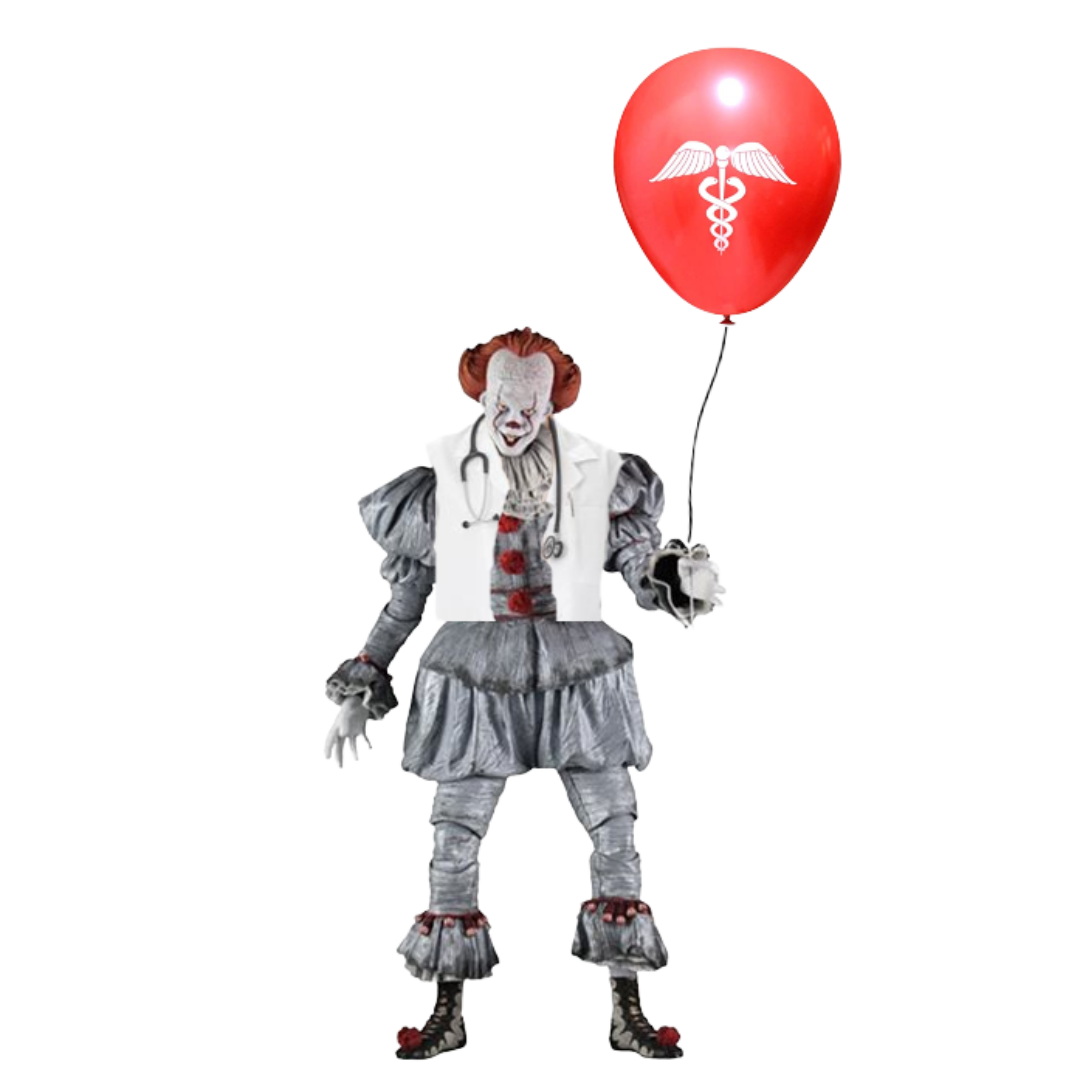
Dicoumarol (Moldy Sweet Clover) Toxicosis in a Group of Holstein Calves
Yamini B, Poppenga RH, Emmett BW, Judge LJ. Dicoumarol (Moldy Sweet Clover) Toxicosis in a Group of Holstein Calves. Journal of Veterinary Diagnostic Investigation. 1995;7(3):420-422. doi:10.1177/104063879500700328
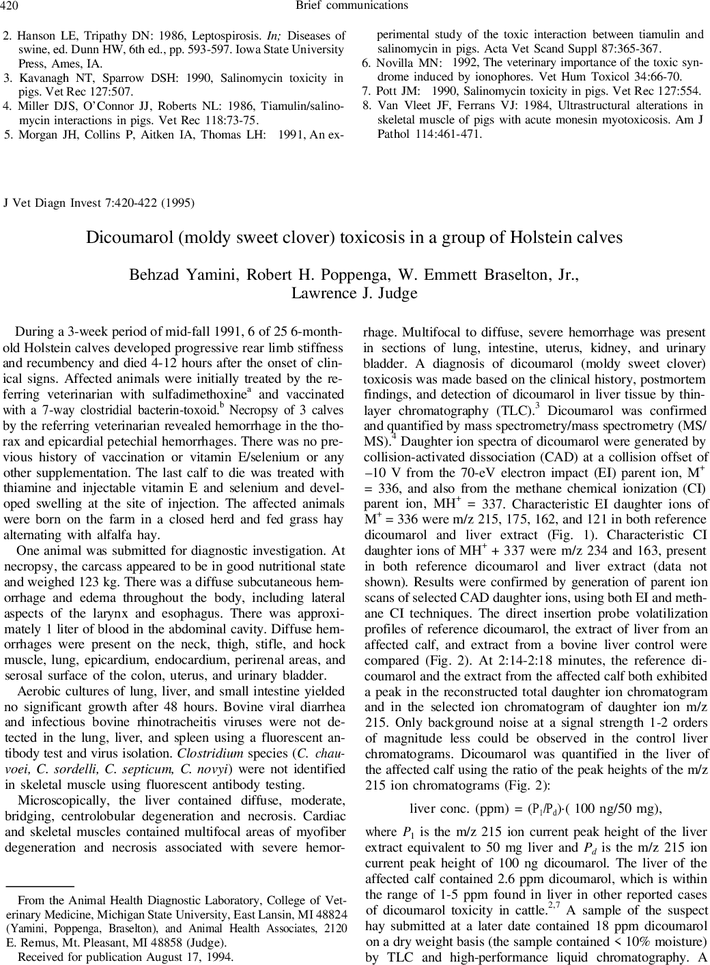
References
1. Alstad AD, Casper HH, Johnson LJ: 1985, Vitamin K treatment of sweet clover poisoning in calves. J Am Vet Med Assoc 187:729–731.
2. Blakley BR: 1985, Moldy sweet clover (dicoumarol) poisoning in Saskatchewan cattle. Can Vet J 26:357–360.
3. Braselton WEJr, Neiger RD, Poppenga RH: 1992, Confirmation of indandione rodenticide toxicosis by mass spectrometry/mass spectrometry. J Vet Diagn Invest 4:441–446.
4. Braselton WEJr, Rumler PC, Poppenga RH: 1992, MS/MS confirmation of hydroxycoumarin toxicosis. Proc Annu Meet Am Assoc Vet Lab Diagn 35:000–000.
5. Benson ME, Casper HH, Johnson LJ: 1981, Occurrence and range of dicoumarol concentrations in sweet clover. Am J Vet Res 42:2014–2015.
6. Casper HH, Alstad AD, Monson SB, Johnson LJ: 1982, Dicoumarol levels in sweet clover toxic to cattle. Proc Annu Meet Am Assoc Vet Lab Diagn 25:000–000.
7. DeHoogh W: 1989, Dicoumarol toxicity in a herd of Ayrshire cattle fed moldy sweet clover. Bovine Pract 24:173–175.
8. Edwards WC, Burrows GE, Tyr RJ: 1984, Toxic plants of Oklahoma: Clovers. Okla Vet Med Assoc 36:30–32.
9. Fraser CM, ed.: 1986, Toxicology In: Merck veterinary manual, 6th ed., pp. 1328–1418. Merck, Rahway, NJ.
10. Goplen BP, Bell JM: 1967, Dicoumarol studies. IV. Antidotal and antagonistic properties of vitamin K1 and K3 in cattle. Can J Anim Sci 47:91–100.
11. Goplen BP, Linton JH, Bell JM: 1964, Dicoumarol studies. III. Determining tolerance limits of contamination in low coumarin sweet clover varieties using a cattle bio-assay. Can J Anim Sci 44:76–86.
12. Osweiler GD, Lawrence PR: 1981, Plants affecting blood coagulation. In: Current veterinary therapy I, food animal practice, ed. Howard JL, pp. 449–451. WB Saunders Co., Philadelphia, PA.
13. Sheel LD: 1978, Mycotoxicosis in cattle. In: Mycotoxic fungi, mycotoxins, mycotoxicosis: an encyclopedic handbook, ed. Wyllie ID, Morehouse LB, pp. 121–142. Dekker, New York, NY.
14. Valli VO: 1985, The hematopoietic system. In: Pathology of domestic animals, ed. Jubb RF, Kennedy CP, Palmer N, 3rd ed., pp. 83–236. Academic Press, New York, NY.
Dicoumarol
Dicoumarol (INN) or dicumarol (USAN) is a naturally occurring anticoagulant drug that depletes stores of vitamin K (similar to warfarin, a drug that dicoumarol inspired). It is also used in biochemical experiments as an inhibitor of reductases.
Dicoumarol is a natural chemical substance of combined plant and fungal origin. It is a derivative of coumarin, a bitter-tasting but sweet-smelling substance made by plants that does not itself affect coagulation, but which is (classically) transformed in mouldy feeds or silages by a number of species of fungi, into active dicoumarol. Dicoumarol does affect coagulation, and was discovered in mouldy wet sweet-clover hay, as the cause of a naturally occurring bleeding disease in cattle.[1] See warfarin for a more detailed discovery history.
Identified in 1940, dicoumarol became the prototype of the 4-hydroxycoumarin anticoagulant drug class. Dicoumarol itself, for a short time, was employed as a medicinal anticoagulant drug, but since the mid-1950s has been replaced by its simpler derivative warfarin, and other 4-hydroxycoumarin drugs. It is given orally, and it acts within two days.
Uses
Dicoumarol was used, along with heparin, for the treatment of deep venous thrombosis. Unlike heparin, this class of drugs may be used for months or years.
Mechanism of action
Like all 4-hydroxycoumarin drugs it is a competitive inhibitor of vitamin K epoxide reductase, an enzyme that recycles vitamin K, thus causing depletion of active vitamin K in blood. This prevents the formation of the active form of prothrombin and several other coagulant enzymes. These compounds are not antagonists of Vitamin K directly—as they are in pharmaceutical uses—but rather promote depletion of vitamin K in bodily tissues allowing vitamin K’s mechanism of action as a potent medication for dicoumarol toxicity. The mechanism of action of Vitamin K along with the toxicity of dicoumarol are measured with the prothrombin time (PT) blood test.
Poisoning
Overdose results in serious, sometimes fatal uncontrolled hemorrhage.[2]
History
Dicoumarol was isolated by Karl Link‘s laboratory at University of Wisconsin, six years after a farmer had brought a dead cow and a milk can full of uncoagulated blood to an agricultural extension station of the university. The cow had died of internal bleeding after eating moldy sweet clover; an outbreak of such deaths had begun in the 1920s during The Great Depression as farmers could not afford to waste hay that had gone bad.[3] Link’s work led to the development of the rat poison warfarin and then to the anticoagulants still in clinical use today.[3]
Footnotes
- Nicole Kresge; Robert D. Simoni; Robert L. Hill (2005-02-25). “Sweet clover disease and warfarin review”. Journal of Biological Chemistry. 280 (8): e5. Retrieved 2012-09-26.
- Duff, I. F.; Shull, W. H. (1949). “Fatal Hemorrhage in Dicumarol® Poisoning”. Journal of the American Medical Association. 139 (12): 762–6. doi:10.1001/jama.1949.02900290008003. PMID 18112552.
- Wardrop, Douglas; Keeling, David (2008). “The story of the discovery of heparin and warfarin” (PDF). British Journal of Haematology. 141 (6): 757–763. doi:10.1111/j.1365-2141.2008.07119.x. PMID 18355382.
See also
Further reading
- Cullen J, Hinkhouse M, Grady M, Gaut A, Liu J, Zhang Y, Weydert C, Domann F, Oberley L (2003). “Dicumarol inhibition of NADPH: quinone oxidoreductase induces growth inhibition of pancreatic cancer via a superoxide-mediated mechanism”. Cancer Res. 63 (17): 5513–20. PMID 14500388.
- Mironov A, Colanzi A, Polishchuk R, Beznoussenko G, Mironov A, Fusella A, Di Tullio G, Silletta M, Corda D, De Matteis M, Luini A (2004). “Dicumarol, an inhibitor of ADP-ribosylation of CtBP3/BARS, fragments golgi non-compact tubular zones and inhibits intra-golgi transport”. Eur J Cell Biol. 83 (6): 263–79. doi:10.1078/0171-9335-00377. PMID 15511084.
- Abdelmohsen K, Stuhlmann D, Daubrawa F, Klotz L (2005). “Dicumarol is a potent reversible inhibitor of gap junctional intercellular communication”. Arch Biochem Biophys. 434 (2): 241–7. doi:10.1016/j.abb.2004.11.002. PMID 15639223.
- Thanos C, Liu Z, Reineke J, Edwards E, Mathiowitz E (2003). “Improving relative bioavailability of dicumarol by reducing particle size and adding the adhesive poly(fumaric-co-sebacic) anhydride”. Pharm Res. 20 (7): 1093–100. doi:10.1023/A:1024474609667. PMID 12880296. S2CID 448086.]
Coumarin
Coumarin or 2H-chromen-2-one is an aromatic organic chemical compound with formula C9H6O2. Its molecule can be described as a benzene molecule with two adjacent hydrogen atoms replaced by a lactone-like chain −(CH)=(CH)−(C=O)−O−, forming a second six-membered heterocycle that shares two carbons with the benzene ring. It can be placed in the benzopyrone chemical class and considered as a lactone. Coumarin is a colorless crystalline solid with a sweet odor resembling the scent of vanilla and a bitter taste. It is found in many plants, where it may serve as a chemical defense against predators. By inhibiting synthesis of vitamin K, a related compound is used as the prescription drug warfarin – an anticoagulant – to inhibit formation of blood clots, deep vein thrombosis, and pulmonary embolism. “Coumarin”. PubChem, National Library of Medicine, US National Institutes of Health. 4 April 2019. Retrieved 13 April 2019. “Coumarins and indandiones”. Drugs.com. 2016. Retrieved 24 December 2016.
Etymology
Coumarin is derived from coumarou, the French word for the tonka bean. The word tonka for the tonka bean is taken from the Galibi (Carib) tongue spoken by natives of French Guiana (one source for the plant); it also appears in Old Tupi, another language of the same region, as the name of the tree. The old genus name, Coumarouna, was formed from another Tupi name for tree, kumarú.
History
Coumarin was first isolated from tonka beans in 1820 by A. Vogel of Munich, who initially mistook it for benzoic acid. Vogel, A. (1820). “Darstellung von Benzoesäure aus der Tonka-Bohne und aus den Meliloten- oder Steinklee-Blumen” [Preparation of benzoic acid from tonka beans and from the flowers of melilot or sweet clover]. Annalen der Physik (in German). 64 (2): 161–166. Bibcode:1820AnP….64..161V. doi:10.1002/andp.18200640205. Vogel, A. (1820). “De l’existence de l’acide benzoïque dans la fève de tonka et dans les fleurs de mélilot” [On the existence of benzoic acid in the tonka bean and in the flowers of melilot]. Journal de Pharmacie (in French). 6: 305–309.
Also in 1820, Nicholas Jean Baptiste Gaston Guibourt (1790–1867) of France independently isolated coumarin, but he realized that it was not benzoic acid.In a subsequent essay he presented to the pharmacy section of the Académie Royale de Médecine, Guibourt named the new substance coumarine. Guibourt, N. J. B. G. (1820). Histoire Abrégée des Drogues Simples [Abridged History of Simple Drugs] (in French). Vol. 2. Paris: L. Colas. pp. 160–161. “Societe du Pharmacie de Paris”. Journal de Chimie Médicale, de Pharmacie et de Toxicologie. 1: 303. 1825. … plus récemment, dans un essai de nomenclature chimique, lu à la section de Pharmacie de l’Académie royale de Médecine, il l’a désignée sous le nom de coumarine, tiré du nom du végétal coumarouna odorata … [… more recently, in an essay on chemical nomenclature, [which was] read to the pharmacy section of the Royal Academy of Medicine, he [Guibourt] designated it by the name “coumarine,” derived from the name of the vegetable Coumarouna odorata …] Guibourt, N. J. B. G. (1869). Histoire Naturelle des Drogues Simples (6th ed.). Paris: J. B. Baillière et fils. p. 377. … la matière cristalline de la fève tonka (matière que j’ai nommée coumarine) … [… the crystalline matter of the tonka bean (matter that I named coumarine …]
In 1835, the French pharmacist A. Guillemette proved that Vogel and Guibourt had isolated the same substance. Coumarin was first synthesized in 1868 by the English chemist William Henry Perkin. Guillemette, A. (1835). “Recherches sur la matière cristalline du mélilot” [Research into the crystalline material of melilot]. Journal de Pharmacie. 21: 172–178. Perkin, W. H. (1868). “On the artificial production of coumarin and formation of its homologues”. Journal of the Chemical Society. 21: 53–63. doi:10.1039/js8682100053.
Coumarin has been an integral part of the fougère genre of perfume since it was first used in Houbigant’s Fougère Royale in 1882. “Olfactory Groups – Aromatic Fougere”. fragrantica.com. Retrieved 13 November 2020.
Synthesis
Coumarin can be prepared by a number of name reactions, with the Perkin reaction between salicylaldehyde and acetic anhydride being a popular example. The Pechmann condensation provides another route to coumarin and its derivatives, as does the Kostanecki acylation, which can also be used to produce chromones.
Biosynthesis
From lactonization of ortho-hydroxylated cis–hydroxycinnamic acid. Jacobowitz, Joseph R.; Weng, Jing-Ke (2020-04-29). “Exploring Uncharted Territories of Plant Specialized Metabolism in the Postgenomic Era”. Annual Review of Plant Biology. Annual Reviews. 71 (1): 631–658. doi:10.1146/annurev-arplant-081519-035634. ISSN 1543-5008. PMID 32176525. S2CID 212740956.
Natural occurrence
Coumarin is found naturally in many plants. Freshly ground plant parts contain higher amount of desired and undesired phytochemicals than powder. Also, whole plant parts are harder to counterfeit; for example, one study showed that authentic Ceylon cinnamon bark contained 0.012 to 0.143 mg/g coumarin, but samples purchased at markets contained up to 3.462 mg/g, possibly because those were mixed with other cinnamon varieties. Ananthakrishnan, R.; Chandra, Preeti; Kumar, Brijesh; Rameshkumar, K. B. (1 January 2018). “Quantification of coumarin and related phenolics in cinnamon samples from south India using UHPLC-ESI-QqQLIT-MS/MS method”. International Journal of Food Properties. 21: 50–57. doi:10.1080/10942912.2018.1437629. S2CID 104289832.
- Vanilla grass (Anthoxanthum odoratum)
- Sweet woodruff (Galium odoratum)
- Sweet grass (Hierochloe odorata)
- Sweet-clover (genus Melilotus)
- Tonka bean (Dipteryx odorata)
- Cinnamon; a 2013 study showed different varieties containing different levels of coumarin:
- Ceylon cinnamon or true cinnamon (Cinnamomum verum): 0.005 to 0.090 mg/g
- Chinese cinnamon or Chinese cassia (C. cassia): 0.085 to 0.310 mg/g
- Indonesian cinnamon or Padang cassia (C. burmannii): 2.14 to 9.30 mg/g
- Saigon cinnamon or Vietnamese cassia (C. loureiroi): 1.06 to 6.97 mg/g
- Deertongue (Carphephorus odoratissimus),
- Tilo (Justicia pectoralis),
- Mullein (genus Verbascum)
- Many cherry blossom tree varieties (of the genus Prunus).
- Related compounds are found in some but not all specimens of genus Glycyrrhiza, from which the root and flavour licorice derives.
Cassia Cinnamon as a Source of Coumarin in Cinnamon-Flavored Food and Food Supplements in the United States J. Agric. Food Chem., 61 (18), 4470–4476 Khan, Ikhlas A.; Ehab, Abourashed A. (2010). Leung’s Encyclopedia of Common Natural Ingredients Used in Food, Drugs, and Cosmetics (PDF). Hoboken, NJ USA: John Wiley & Sons. pp. 240–242. ISBN 978-9881607416. Retrieved 21 September 2020. Leal, L. K. A. M.; Ferreira, A. A. G.; Bezerra, G. A.; Matos, F. J. A.; Viana, G. S. B. (May 2000). “Antinociceptive, anti-inflammatory and bronchodilator activities of Brazilian medicinal plants containing coumarin: a comparative study”. Journal of Ethnopharmacology. 70 (2): 151–159. doi:10.1016/S0378-8741(99)00165-8. ISSN 0378-8741. PMID 10771205. Lino, C. S.; Taveira, M. L.; Viana, G. S. B.; Matos, F. J. A. (1997). “Analgesic and antiinflammatory activities of Justicia pectoralis Jacq. and its main constituents: coumarin and umbelliferone”. Phytotherapy Research. 11 (3): 211–215. doi:10.1002/(SICI)1099-1573(199705)11:3<211::AID-PTR72>3.0.CO;2-W. S2CID 84525194. Archived from the original on 2013-01-05. Retrieved 2010-06-26. Ieri, Francesca; Pinelli, Patrizia; Romani, Annalisa (2012). “Simultaneous determination of anthocyanins, coumarins and phenolic acids in fruits, kernels and liqueur of Prunus mahaleb L”. Food Chemistry. 135 (4): 2157–2162. doi:10.1016/j.foodchem.2012.07.083. hdl:2158/775163. PMID 22980784. Hatano, T.; et al. (1991). “Phenolic constituents of licorice. IV. Correlation of phenolic constituents and licorice specimens from various sources, and inhibitory effects of…” Yakugaku Zasshi. 111 (6): 311–21. doi:10.1248/yakushi1947.111.6_311. PMID 1941536.
Coumarin is found naturally also in many edible plants such as strawberries, black currants, apricots, and cherries. “Coumarin”. PubChem, National Library of Medicine, US National Institutes of Health. 4 April 2019. Retrieved 13 April 2019.
Coumarins were found to be uncommon but occasional components of propolis by Santos-Buelga and Gonzalez-Paramas 2017. Berenbaum, May R.; Calla, Bernarda (2021-01-07). “Honey as a Functional Food for Apis mellifera”. Annual Review of Entomology. Annual Reviews. 66 (1): 185–208. doi:10.1146/annurev-ento-040320-074933. ISSN 0066-4170. PMID 32806934. S2CID 221165130.
Biological function
Coumarin has appetite-suppressing properties, which may discourage animals from eating plants that contain it. Though the compound has a pleasant sweet odor, it has a bitter taste, and animals tend to avoid it. Link, K. P. (1 January 1959). “The discovery of dicumarol and its sequels”. Circulation. 19 (1): 97–107. doi:10.1161/01.CIR.19.1.97. PMID 13619027.
Metabolism
The biosynthesis of coumarin in plants is via hydroxylation, glycolysis, and cyclization of cinnamic acid.[citation needed] In humans, the enzyme encoded by the gene UGT1A8 has glucuronidase activity with many substrates, including coumarins. Ritter, J. K.; et al. (Mar 1992). “A novel complex locus UGT1 encodes human bilirubin, phenol, and other UDP-glucuronosyltransferase isozymes with identical carboxyl termini”. J. Biol. Chem. 267 (5): 3257–3261. doi:10.1016/S0021-9258(19)50724-4. PMID 1339448
- UDP-glucuronosyltransferase 1-8 is an enzyme that in humans is encoded by the UGT1A8 gene. This gene encodes a UDP-glucuronosyltransferase, an enzyme of the glucuronidation pathway that transforms small lipophilic molecules, such as steroids, bilirubin, hormones, and drugs, into water-soluble, excretable metabolites. This gene is part of a complex locus that encodes several UDP-glucuronosyltransferases. The locus includes thirteen unique alternate first exons followed by four common exons. Four of the alternate first exons are considered pseudogenes. Each of the remaining nine 5′ exons may be spliced to the four common exons, resulting in nine proteins with different N-termini and identical C-termini. Each first exon encodes the substrate binding site, and is regulated by its own promoter. The enzyme encoded by this gene has glucuronidase activity with many substrates including coumarins, phenols, anthraquinones, flavones, and some opioids. GRCh38: Ensembl release 89: ENSG00000242366 – Ensembl, May 2017 GRCm38: Ensembl release 89: ENSMUSG00000090175 – Ensembl, May 2017 “Human PubMed Reference:”. National Center for Biotechnology Information, U.S. National Library of Medicine. “Mouse PubMed Reference:”. National Center for Biotechnology Information, U.S. National Library of Medicine. Ritter JK, Chen F, Sheen YY, Tran HM, Kimura S, Yeatman MT, Owens IS (Mar 1992). “A novel complex locus UGT1 encodes human bilirubin, phenol, and other UDP-glucuronosyltransferase isozymes with identical carboxyl termini”. J Biol Chem. 267 (5): 3257–61. doi:10.1016/S0021-9258(19)50724-4. PMID 1339448. Huang YH, Galijatovic A, Nguyen N, Geske D, Beaton D, Green J, Green M, Peters WH, Tukey RH (June 2002). “Identification and functional characterization of UDP-glucuronosyltransferases UGT1A81, UGT1A82 and UGT1A8*3″. Pharmacogenetics. 12 (4): 287–97. doi:10.1097/00008571-200206000-00004. PMID 12042666. “Entrez Gene: UGT1A8 UDP glucuronosyltransferase 1 family, polypeptide A8”.
Related compounds and derivatives
Coumarin and its derivatives are all considered phenylpropanoids.
Some naturally occurring coumarin derivatives include umbelliferone (7-hydroxycoumarin), aesculetin (6,7-dihydroxycoumarin), herniarin (7-methoxycoumarin), psoralen and imperatorin.
4-Phenylcoumarin is the backbone of the neoflavones, a type of neoflavonoids.
Coumarin pyrazole hybrids have been synthesized from hydrazones, carbazones and thiocarbazones via Vilsmeier Haack formylation reaction.
Main article: 4-Hydroxycoumarins
Compounds derived from coumarin are also called coumarins or coumarinoids; this family includes:
- brodifacoum
- Brodifacoum is a highly lethal 4-hydroxycoumarin vitamin K antagonist anticoagulant poison. In recent years, it has become one of the world’s most widely used pesticides. It is typically used as a rodenticide, but is also used to control larger pests such as possum. Brodifacoum has an especially long half-life in the body, which ranges up to nine months, requiring prolonged treatment with antidotal vitamin K for both human and pet poisonings. It has one of the highest risks of secondary poisoning to both mammals and birds. Significant experience in brodifacoum poisonings has been gained in many human cases where it has been used in attempted suicides, necessitating long periods of vitamin K treatment. In March 2018, cases of severe coagulopathy and bleeding associated with synthetic cannabinoid use contaminated with brodifacoum were reported in five states of the US. Brodifacoum is a 4-hydroxycoumarin anticoagulant, with a similar mode of action to its historical predecessors dicoumarol and warfarin. However, due to very high potency and long duration of action (elimination half-life of 20 – 130 days), it is characterised as a “second-generation” or “superwarfarin” anticoagulant. Brodifacoum inhibits the enzyme vitamin K epoxide reductase, which is needed for the reconstitution of the vitamin K in its cycle from vitamin K-epoxide, so brodifacoum steadily decreases the level of active vitamin K in the blood. Vitamin K is required for the synthesis of important substances including prothrombin, which is involved in blood clotting. This disruption becomes increasingly severe until the blood effectively loses any ability to clot. In addition, brodifacoum (as with other anticoagulants in toxic doses) increases permeability of blood capillaries; the blood plasma and blood itself begin to leak from the smallest blood vessels. A poisoned animal suffers progressively worsening internal bleeding, leading to shock, loss of consciousness, and eventually death.[citation needed] Brodifacoum is highly lethal to mammals and birds, and extremely lethal to fish. It is a highly cumulative poison, due to its high lipophilicity and extremely slow elimination.[citation needed] Merck Index, 11th Edition, 1368 Eason, C.T. and Wickstrom, M. Vertebrate pesticide toxicology manual, New Zealand Department of Conservation Rodenticides: Topic Fact Sheet, National Pesticide Information Center van Heerden P. S.; Bezuidenhoudt B. C. (1997), “Efficient Asymmetric Synthesis of the Four Diastereomers of Diphenacoum and Brodifacoum”, Tetrahedron, 43 (17): 6045–6056, doi:10.1016/S0040-4020(97)00254-8 “Brodifacoum (HSG 93, 1995)”. Inchem.org. Retrieved 2013-12-08.
- bromadiolone
- Bromadiolone is a potent anticoagulant rodenticide. It is a second-generation 4-hydroxycoumarin derivative and vitamin K antagonist, often called a “super-warfarin” for its added potency and tendency to accumulate in the liver of the poisoned organism. When first introduced to the UK market in 1980, it was effective against rodent populations that had become resistant to first generation anticoagulants. It is classified as an extremely hazardous substance in the United States as defined in Section 302 of the Emergency Planning and Community Right-to-Know Act (42 U.S.C. 11002), and is subject to strict reporting requirements by facilities which produce, store, or use it in significant quantities. Bromadiolone can be absorbed through the digestive tract, through the lungs, or through skin contact. The pesticide is generally given orally. The substance is a vitamin K antagonist. The lack of vitamin K in the circulatory system reduces blood clotting and will cause death due to internal hemorrhaging. Poisoning does not show effects for 24 to 36 hours after it is eaten and can take up to 2–5 days to cause death. The compound is used as a mixture of four stereoisomers. Its two stereoisomeric centers are at the phenyl- and the hydroxyl-substituted carbons in the carbon chain of the substituent at the 3 position of the coumarin. “40 C.F.R.: Appendix A to Part 355—The List of Extremely Hazardous Substances and Their Threshold Planning Quantities” (PDF) (July 1, 2008 ed.). Government Printing Office. Archived from the original (PDF) on February 25, 2012. Retrieved October 29, 2011. Bromadiolone Archived December 21, 2006, at the Wayback Machine “The Veterinarian’s Guide to accidental rodenticide ingestion by dogs & cats” (PDF). Archived from the original (PDF) on 2016-07-09. Retrieved 2016-08-03. “Bromadiolone (Bromone, Maki) Chemical Profile 1/85”. Pesticide Management Education Program, Cornell University. Archived from the original on 2019-09-04. Retrieved 2021-05-24.
- difenacoum
- Difenacoum is an anticoagulant of the 4-hydroxycoumarinvitamin K antagonist type. It has anticoagulant effects and is used commercially as a rodenticide. It was first introduced in 1976 and first registered in the USA in 2007. Difenacoum is sold as blue-green and red pellets. Difenacoum was first introduced in 1976 as a rodenticide effective against rats and mice which were resistant to other anticoagulants. Vitamin K reverses the anticoagulant effect of rodenticides over a period of 24 to 48 hours from initiation of therapy. Caught early enough, Vitamin K can be rapidly administered by subcutaneous injection and followed up with by food-based supplements. Because other species of mammals and birds may prey upon affected rodents, or directly ingest rodenticide bait, there is a risk of primary, secondary or tertiary exposure; examples are described in a 2012 publication on veterinary toxicology. Using radiolabeled isotopes, difenacoum (and/or its metabolites) has been shown to be distributed across many organ tissues upon oral ingestion, with the highest concentrations occurring in the liver and pancreas. Difenacoum has been shown to be highly toxic to some species of freshwater fish and green algae despite the fact that difenacoum is weakly soluble in aqueous solutions. Vitamin K deficiency in animals is deliberately brought about by anticoagulant rodenticide toxicities. Vitamin K deficiency causes internal bleeding and hemorrhaging, resulting in a slow, painful death. Other vitamin K deficient states include: biliary obstruction, intrahepatic cholestasis, intestinal malabsorption and chronic oral antibiotic administration. These are objectives of these poisons, as the sick animal normally stays in its nest, removing the need to clean up/dispose of dead animals. Often the target animals will remain healthy enough to feed several times and possibly bring poisoned foods back to feed their young. The poison is effectively transferred through milk of mothers to nursing mammalian infants. “EPA: Difenacoum”. Retrieved 3 April 2015.[permanent dead link] “University of Hertfordshire: IUPAC: difenacoum”. Retrieved 3 April 2015. Gupta, Ramesh C. (ed) (2012). Veterinary Toxicology: Basic and clinical principles. Academic Press. p. 673-697. ISBN 9780123859273. Retrieved 3 April 2015. “Vitamin K Therapy”. 8 February 2019.
- auraptene
- Auraptene is a natural bioactive monoterpene coumarin ether. It was first isolated from members of the genus Citrus. Auraptene has shown some effect as a chemopreventative agent against cancers of liver, skin, tongue, esophagus, and colon in rodent models. Curini, M., Carvotto, G., Epifano, F. and Giannone, G. “Chemistry and Biological Activity of Natural and Synthetic Prenyloxycoumarins”(2006). Current Medicinal Chemistry, 13, 199-222.
- ensaculin
- Ensaculin (KA-672) is a drug from the coumarin family, which has been researched as a potential treatment for dementia. It acts on a number of receptor systems, being both a weak NMDA antagonist and a 5HT1A agonist. Animal studies have shown promising nootropic effects, although efficacy in humans has yet to be proven. It was well tolerated in human trials, with the main side effect being orthostatic hypotension (low blood pressure). Lishko PV, Maximyuk OP, Chatterjee SS, Nöldner M, Krishtal OA (December 1998). “The putative cognitive enhancer KA-672.HCl is an uncompetitive voltage-dependent NMDA receptor antagonist”. NeuroReport. 9 (18): 4193–7. doi:10.1097/00001756-199812210-00035. PMID 9926872. S2CID 29960822. Winter JC, Helsley SE, Rabin RA (July 1998). “The discriminative stimulus effects of KA 672, a putative cognitive enhancer: evidence for a 5-HT1A component”. Pharmacology, Biochemistry, and Behavior. 60 (3): 703–7. doi:10.1016/S0091-3057(98)00043-4. PMID 9678654. S2CID 6493994. Hoerr R, Noeldner M (2002). “Ensaculin (KA-672 HCl): a multitransmitter approach to dementia treatment”. CNS Drug Reviews. 8 (2): 143–58. doi:10.1111/j.1527-3458.2002.tb00220.x. PMC 6741668. PMID 12177685. Knauber J, Müller WE (March 2003). “Anseculin improves passive avoidance learning of aged mice”. Pharmacological Research. 47 (3): 225–33. doi:10.1016/S1043-6618(02)00311-0. PMID 12591018. Sourgens H, Hoerr R, Biber A, Steinbrede H, Derendorf H (April 1998). “KA 672-HCl, a neuronal activator against dementia: tolerability, safety, and preliminary pharmacokinetics after single and multiple oral doses in healthy male and female volunteers”. Journal of Clinical Pharmacology. 38 (4): 373–81. doi:10.1002/j.1552-4604.1998.tb04438.x. PMID 9590466. S2CID 32558296.
- phenprocoumon (Marcoumar)
- Phenprocoumon (marketed under the brand names Marcoumar, Marcumar and Falithrom) is a long-acting blood thinner drug to be taken by mouth, and a derivative of coumarin. It acts as a vitamin K antagonist and inhibits blood clotting (coagulation) by blocking synthesis of coagulation factors II, VII, IX and X. It is used for the prophylaxis and treatment of thromboembolic disorders such as heart attacks and pulmonary (lung) embolism. The most common adverse effect is bleeding. The drug interacts with a large number of other medications, including aspirin and St John’s Wort. It is the standard coumarin used in Germany, Austria, and other European countries. Phenprocoumon is contraindicated when bleeding risks exceed the potential benefits, for example in people with severe bleeding diathesis, peptic ulcers, endocarditis, aortic aneurysm, brain aneurysm, serious injuries, or after brain surgery. During pregnancy, it is contraindicated except to prevent coagulation in women with life-threatening heparin intolerance. The most common adverse effect is bleeding. It occurs in 5–25% of patients and ranges from harmless nosebleeds to life-threatening bleeding in the brain, gut wall, adrenal glands, pleural cavity, pericardium, or subdural space. Other side effects are uncommon and include headache, nausea, reversible hair loss, purple toe syndrome, and allergic rashes. A rare but severe adverse effect is warfarin necrosis of the skin and subcutaneous tissue during the first days of treatment. Mild cases of overdosing are characterised by minor bleeding and/or bruising; they can usually be controlled by reducing the dose. Taking a large amount of phenprocoumon at once can lead to brain edema during the first 24 hours, followed by reduced blood clotting and (possibly severe) bleeding, including blood in the stool or urine. Bleeding into the brain can lead to disorientation or unconsciousness, calling for immediate medical intervention. Overdosing for an extended time period is toxic for the liver parenchyma, the kidneys’ glomeruli, and blood vessels.
- Due to its narrow therapeutic index, the fact that it can only be eliminated from the body after inactivation by the liver enzymes CYP2C9 and CYP3A4, and its high plasma protein binding (see below), phenprocoumon has significant interactions with a large number of other drugs and with some kinds of food. Some examples are:
- CYP2C9 inducers decrease its concentrations and therefore its anticoagulant effect: rifampicin, St John’s wort.
- CYP3A4 inducers also decrease its anticoagulant effect: also rifampicin and St John’s wort.
- CYP2C9 inhibitors increase its concentrations and therefore the risk for bleeding: amiodarone.
- CYP3A4 inhibitors also increase the risk for bleeding: clarithromycin, ketoconazole, grapefruit juice, also amiodarone.
- Substances that displace phenprocoumon from its plasma protein binding increase its free blood concentrations and therefore increase the risk for bleeding: a number of nonsteroidal anti-inflammatory drugs (NSAIDs), especially aspirin.
- Antibiotics increase the risk for bleeding, probably because they kill vitamin K producing bacteria of the gut flora: possibly all antibiotics; documented for amoxicillin and quinolone antibiotics among others.
- Food containing vitamin K, such as cabbage, spinach and broccoli, might reduce the drug’s effectivity. High doses of vitamin K antagonise phenprocoumon 20 to 24 hours after ingestion or infusion; the effect lasts for 7 to 10 days.
- Phenprocoumon is an inhibitor of the enzyme vitamin K epoxide reductase (VKOR). Vitamin K is needed to activate the coagulation factors II, VII, IX and X and the anticoagulation factors protein C and protein S, in which process it turns into vitamin K 2,3-epoxide. This is then recycled to vitamin K in a process involving VKOR. Inhibiting this enzyme effectively creates a vitamin K deficiency, blocking activation of the coagulation factors. After 36 to 72 hours, the available activated factors have been depleted (used up) by the coagulation system, and the anticoagulation takes effect. The drug is taken by mouth and quickly and completely absorbed from the gut. When in the bloodstream, 99% are bound to plasma proteins (mainly albumin). The substance is metabolized by the liver enzymes CYP2C9 and CYP3A4 to various hydroxyl derivatives, and subsequently conjugated to glucuronic acid to a small extent. The glucuronide metabolites partly undergo enterohepatic circulation. All metabolites, as well as the parent substance, are excreted predominantly via the kidney and urine, only 15% in unchanged form. The terminal half-life is 150 hours (6 to 7 days) on average with large differences between people. The long half-life means that drug concentrations take about four weeks to reach a steady state after therapy is started; the anticoagulation lasts at least 7 to 10 days after therapy is stopped; and dose changes need several days to take effect. The drug passes the placenta and also into breast milk. Dinnendahl V, Fricke U, eds. (2000). Arzneistoff-Profile (in German). Vol. 8 (16 ed.). Eschborn, Germany: Govi Pharmazeutischer Verlag. Doxycyclin. ISBN 978-3-7741-9846-3. Haustein KO (1999). “Pharmacokinetic and pharmacodynamic properties of oral anticoagulants, especially phenprocoumon”. Seminars in Thrombosis and Hemostasis. 25 (1): 5–11. doi:10.1055/s-2007-996417. PMID 10327214. Geisslinger G, Menzel S (15 September 2017). Wenn Arzneimittel wechselwirken (in German). Stuttgart: Wissenschaftliche Verlagsgesellschaft. pp. 15–18. ISBN 978-3-8047-3747-1 Haberfeld H, ed. (2021). Austria-Codex (in German). Vienna: Österreichischer Apothekerverlag. Marcoumar-Tabletten. Text available here. Phenprocoumon International Drug Names. Accessed 2021-08-25. “mediQ (paywalled)” (in German). Psychiatrische Dienste Aargau AG. Retrieved 2021-08-24. Nöldeke S, Jenal G, Hupp T (2001). “Coumarin-induced necrosis: Pathophysiology, clinical picture, and therapy”. Gefässchirurgie. 6 (3): 129–135. doi:10.1007/s007720100143. S2CID 44842896. Karow T, Lang-Roth R (2021). Pharmakologie und Toxikologie (in German) (29 ed.). pp. 161–163. ISBN 9783982122311. Mutschler E, Schäfer-Korting M (2013). Arzneimittelwirkungen (in German) (10 ed.). Stuttgart: Wissenschaftliche Verlagsgesellschaft. pp. 481–2. ISBN 978-3-8047-1763-3. Castoldi E, Hackeng TM (September 2008). “Regulation of coagulation by protein S”. Curr. Opin. Hematol. 15 (5): 529–36. doi:10.1097/MOH.0b013e328309ec97. PMID 18695379. S2CID 11522770. Kammerer B, Kahlich R, Ufer M, Schenkel A, Laufer S, Gleiter CH (November 2005). “Stereospecific pharmacokinetic characterisation of phenprocoumon metabolites, and mass-spectrometric identification of two novel metabolites in human plasma and liver microsomes”. Analytical and Bioanalytical Chemistry. 383 (6): 909–17. doi:10.1007/s00216-005-0113-7. PMID 16237546. S2CID 13730692. Kammerer B, Kahlich R, Ufer M, Schenkel A, Laufer S, Gleiter CH (November 2005). “Stereospecific pharmacokinetic characterisation of phenprocoumon metabolites, and mass-spectrometric identification of two novel metabolites in human plasma and liver microsomes”. Analytical and Bioanalytical Chemistry. 383 (6): 909–17. doi:10.1007/s00216-005-0113-7. PMID 16237546. S2CID 13730692.
- PSB-SB-487
- PSB-SB-487 is a coumarin derivative which is an antagonist at the former orphan receptorGPR55. Unlike older GPR55 antagonists such as O-1918, PSB-SB-487 has good selectivity over the related receptor GPR18, with an IC50 of 113nM at GPR55 vs 12500nM at GPR18. However it has poorer selectivity over other related receptors, acting as a weak antagonist at CB1 with a Ki of 1170nM, and a partial agonist at CB2 with a Ki of 292nM. Rempel V, Volz N, Gläser F, Nieger M, Bräse S, Müller CE (June 2013). “Antagonists for the orphan G-protein-coupled receptor GPR55 based on a coumarin scaffold”. Journal of Medicinal Chemistry. 56 (11): 4798–810. doi:10.1021/jm4005175. PMID 23679955. Rempel V, Volz N, Hinz S, Karcz T, Meliciani I, Nieger M, et al. (September 2012). “7-Alkyl-3-benzylcoumarins: a versatile scaffold for the development of potent and selective cannabinoid receptor agonists and antagonists”. Journal of Medicinal Chemistry. 55 (18): 7967–77. doi:10.1021/jm3008213. PMID 22916707.
- PSB-SB-1202
- PSB-SB-1202 is a coumarin derivative which is an agonist at the cannabinoid receptors CB1 and CB2, with a CB1 Ki of 32nM and a CB2 Ki of 49nM. It is also a weak antagonist at the related receptor GPR55, with an IC50 of 6350nM, but has no significant affinity for GPR18. Rempel V, Volz N, Hinz S, Karcz T, Meliciani I, Nieger M, et al. (September 2012). “7-Alkyl-3-benzylcoumarins: a versatile scaffold for the development of potent and selective cannabinoid receptor agonists and antagonists”. Journal of Medicinal Chemistry. 55 (18): 7967–77. doi:10.1021/jm3008213. PMID 22916707. Rempel V, Volz N, Gläser F, Nieger M, Bräse S, Müller CE (June 2013). “Antagonists for the orphan G-protein-coupled receptor GPR55 based on a coumarin scaffold”. Journal of Medicinal Chemistry. 56 (11): 4798–810. doi:10.1021/jm4005175. PMID 23679955.
- Scopoletin can be isolated from the bark of Shorea pinanga
- Scopoletin is a coumarin. It found in the root of plants in the genus Scopolia such as Scopolia carniolica and Scopolia japonica, in chicory, in Artemisia scoparia, in the roots and leaves of stinging nettle (Urtica dioica), in the passion flower, in Brunfelsia, in Viburnum prunifolium, in Solanum nigrum, in Datura metel, in Mallotus resinosus, or and in Kleinhovia hospita. It can also be found in fenugreek, vinegar, some whiskies or in dandelion coffee. A similar coumarin is scoparone. Scopoletin is highly fluorescent when dissolved in DMSO or water and is regularly used as a fluorimetric assay for the detection of hydrogen peroxide in conjunction with horseradish peroxidase. When oxidized, its fluorescence is strongly suppressed. Like most phenylpropanoids, the biosynthetic precursor to scopoletin acid is 4-coumaroyl-CoA. Scopoletin is derived from 1,2-benzopyrones which is the core structure of coumarins formed through hydroxylation of cinnamates, trans/cis isomerization of the side chain, and lactonization. And CYP98A (C3’H) are enzymes belonging to the cytochrome P450 family that catalyze the meta-hydroxylation of p-coumarate derivatives, an important step in the phenylpropanoid pathway. For scopoletin, most of biosynthetic investigations are based on Arabidopsis thaliana. Zhao Y; Liu F; Lou HX (2010). “[Studies on the chemical constituents of Solanum nigrum]”. Zhong Yao Cai (in Chinese). 33 (4): 555–556. PMID 20845784. Han XL, Wang H, Zhang ZH, Tan Y, Wang JH (August 2015). “[Study on Chemical Constituents in Seeds of Datura metel from Xinjiang]”. Zhong Yao Cai = Zhongyaocai = Journal of Chinese Medicinal Materials (in Chinese). 38 (8): 1646–8. PMID 26983236. Ma J; Jones SH; Hecht SM (2004). “A coumarin from Mallotus resinosus that mediates DNA cleavage”. J Nat Prod. 67 (9): 1614–1616. doi:10.1021/np040129c. PMID 15387675. Ouzir, M; El Bairi, K; Amzazi, S (October 2016). “Toxicological properties of fenugreek (Trigonella foenum graecum)”. Food and Chemical Toxicology. 96: 145–54. doi:10.1016/j.fct.2016.08.003. PMID 27498339. Analysis of polyphenolic compounds of different vinegar samples. Miguel Carrero Gálvez, Carmelo García Barroso and Juan Antonio Pérez-Bustamante, Zeitschrift für Lebensmitteluntersuhung und -Forschung A, Volume 199, Number 1, pages 29-31, doi:10.1007/BF01192948 Vogt, T. (2010). “Phenylpropanoid Biosynthesis”. Molecular Plant. 3: 2–20. doi:10.1093/mp/ssp106. PMID 20035037. Beeching, John R.; Han, Yuanhuai; Gómez-Vásquez, Rocío; Day, Robert C.; Cooper, Richard M. (1998), “Wound and Defense Responses in Cassava as Related to Post-Harvest Physiological Deterioration”, Phytochemical Signals and Plant—Microbe Interactions, Springer US, pp. 231–248, doi:10.1007/978-1-4615-5329-8_12, ISBN 9780306459177 Kai, Kosuke; Mizutani, Masaharu; Kawamura, Naohiro; Yamamoto, Ryotaro; Tamai, Michiko; Yamaguchi, Hikaru; Sakata, Kanzo; Shimizu, Bun-ichi (September 2008). “Scopoletin is biosynthesized viaortho-hydroxylation of feruloyl CoA by a 2-oxoglutarate-dependent dioxygenase inArabidopsis thaliana”. The Plant Journal. 55 (6): 989–999. doi:10.1111/j.1365-313x.2008.03568.x. ISSN 0960-7412. PMID 18547395. Schoch, Guillaume; Goepfert, Simon; Morant, Marc; Hehn, Alain; Meyer, Denise; Ullmann, Pascaline; Werck-Reichhart, Danièle (2001-06-27). “CYP98A3 fromArabidopsis thalianaIs a 3′-Hydroxylase of Phenolic Esters, a Missing Link in the Phenylpropanoid Pathway” (PDF). Journal of Biological Chemistry. 276 (39): 36566–36574. doi:10.1074/jbc.m104047200. ISSN 0021-9258. PMID 11429408. S2CID 11765327.
- warfarin (Coumadin)
International Programme on Chemical Safety. “Brodifacoum (pesticide data sheet)”. Archived from the original on 2006-12-09. Retrieved 2006-12-14. Laposata, M; Van Cott, E. M.; Lev, M. H. (2007). “Case 1-2007—A 40-Year-Old Woman with Epistaxis, Hematemesis, and Altered Mental Status”. New England Journal of Medicine. 356 (2): 174–82. doi:10.1056/NEJMcpc069032. PMID 17215536. International Programme on Chemical Safety. “Bromadiolone (pesticide data sheet)”. Archived from the original on 2006-12-21. Retrieved 2006-12-14. International Programme on Chemical Safety. “Difenacoum (health and safety guide)”. Retrieved 2006-12-14. Syah, Y. M.; et al. (2009). “A modified oligostilbenoid, diptoindonesin C, from Shorea pinanga Scheff”. Natural Product Research. 23 (7): 591–594. doi:10.1080/14786410600761235. PMID 19401910. S2CID 20216115.
Coumarin is transformed into the natural anticoagulant dicoumarol by a number of species of fungi. This occurs as the result of the production of 4-hydroxycoumarin, then further (in the presence of naturally occurring formaldehyde) into the actual anticoagulant dicoumarol, a fermentation product and mycotoxin. Dicoumarol was responsible for the bleeding disease known historically as “sweet clover disease” in cattle eating moldy sweet clover silage. Venugopala, K. N.; Rashmi, V; Odhav, B (2013). “Review on Natural Coumarin Lead Compounds for Their Pharmacological Activity”. BioMed Research International. 2013: 1–14. doi:10.1155/2013/963248. PMC 3622347. PMID 23586066. Bye, A.; King, H. K. (1970). “The biosynthesis of 4-hydroxycoumarin and dicoumarol by Aspergillus fumigatus Fresenius”. Biochemical Journal. 117 (2): 237–45. doi:10.1042/bj1170237. PMC 1178855. PMID 4192639.
Toxicity
Coumarin is moderately toxic to the liver and kidneys of rodents, with a median lethal dose (LD50) of 293 mg/kg in the rat, a low toxicity compared to related compounds. Coumarin is hepatotoxic in rats, but less so in mice. Rodents metabolize it mostly to 3,4-coumarin epoxide, a toxic, unstable compound that on further differential metabolism may cause liver cancer in rats and lung tumors in mice. Humans metabolize it mainly to 7-hydroxycoumarin, a compound of lower toxicity, and no adverse affect has been directly measured in humans. The German Federal Institute for Risk Assessment has established a tolerable daily intake (TDI) of 0.1 mg coumarin per kg body weight, but also advises that higher intake for a short time is not dangerous. The Occupational Safety and Health Administration (OSHA) of the United States does not classify coumarin as a carcinogen for humans. Coumarin Material Safety Data Sheet (MSDS) Archived 2004-10-21 at the Wayback Machine
Vassallo, J. D.; et al. (2004). “Metabolic detoxification determines species differences in coumarin-induced hepatotoxicity”. Toxicological Sciences. 80 (2): 249–57. doi:10.1093/toxsci/kfh162. PMID 15141102. Born, S. L.; et al. (2003). “Comparative metabolism and kinetics of coumarin in mice and rats”. Food and Chemical Toxicology. 41 (2): 247–58. doi:10.1016/s0278-6915(02)00227-2. PMID 12480300. Lake, B.G (1999). “Coumarin Metabolism, Toxicity and Carcinogenicity: Relevance for Human Risk Assessment”. Food and Chemical Toxicology. 37 (4): 423–453. doi:10.1016/S0278-6915(99)00010-1. PMID 10418958. “Frequently Asked Questions about coumarin in cinnamon and other foods” (PDF). The German Federal Institute for Risk Assessment. 30 October 2006. Archived from the original (PDF) on 19 April 2009. “Chemical Sampling Information – Coumarin”. Osha.gov. Retrieved 30 December 2015.
European health agencies have warned against consuming high amounts of cassia bark, one of the four main species of cinnamon, because of its coumarin content. According to the German Federal Institute for Risk Assessment (BFR), 1 kg of (cassia) cinnamon powder contains about 2.1 to 4.4 g of coumarin. Powdered cassia cinnamon weighs 0.56 g/cm3, so a kilogram of cassia cinnamon powder equals 362.29 teaspoons. One teaspoon of cassia cinnamon powder therefore contains 5.8 to 12.1 mg of coumarin, which may be above the tolerable daily intake value for smaller individuals. However, the BFR only cautions against high daily intake of foods containing coumarin. Its report specifically states that Ceylon cinnamon (Cinnamomum verum) contains “hardly any” coumarin. “Cassia cinnamon with high coumarin contents to be consumed in moderation – BfR”. Bfr.bund.de. Retrieved 30 December 2015. “German Christmas Cookies Pose Health Danger”. NPR.org. 25 December 2006. Retrieved 30 December 2015. “High daily intakes of cinnamon: Health risk cannot be ruled out. BfR Health Assessment No. 044/2006, 18 August 2006” (PDF). bund.de. Retrieved 28 March 2018. Engineering Resources – Bulk Density Chart Archived 2002-10-27 at the Wayback Machine
The European Regulation (EC) No 1334/2008 describes the following maximum limits for coumarin: 50 mg/kg in traditional and/or seasonal bakery ware containing a reference to cinnamon in the labeling, 20 mg/kg in breakfast cereals including muesli, 15 mg/kg in fine bakery ware, with the exception of traditional and/or seasonal bakery ware containing a reference to cinnamon in the labeling, and 5 mg/kg in desserts.
An investigation from the Danish Veterinary and Food Administration in 2013 shows that bakery goods characterized as fine bakery ware exceeds the European limit (15 mg/kg) in almost 50% of the cases. The paper also mentions tea as an additional important contributor to the overall coumarin intake, especially for children with a sweet habit. Ballin, Nicolai Z.; Sørensen, Ann T. (April 2014). “Coumarin content in cinnamon containing food products on the Danish market”. Food Control. 38 (2014): 198–203. doi:10.1016/j.foodcont.2013.10.014.
Coumarin was banned as a food additive in the United States in 1954, largely because of the hepatotoxicity results in rodents. Coumarin is currently listed by the Food and Drug Administration (FDA) of the United States among “Substances Generally Prohibited From Direct Addition or Use as Human Food,” according to 21 CFR 189.130, but some natural additives containing coumarin, such as the flavorant sweet woodruff are allowed “in alcoholic beverages only” under 21 CFR 172.510. In Europe, popular examples of such beverages are Maiwein, white wine with woodruff, and Żubrówka, vodka flavoured with bison grass. Marles, R. J.; et al. (1986). “Coumarin in vanilla extracts: Its detection and significance”. Economic Botany. 41 (1): 41–47. doi:10.1007/BF02859345. S2CID 23232507.
“Food and Drugs”. Access.gpo.gov. Archived from the original on 5 February 2012. Retrieved 30 December 2015.
“FDA/CFSAN/OPA: EAFUS List”. www.cfsan.fda.gov. Archived from the original on 3 September 2000. Retrieved 17 January 2022.
Coumarin is subject to restrictions on its use in perfumery, as some people may become sensitized to it, however the evidence that coumarin can cause an allergic reaction in humans is disputed. “Standards Restricted – IFRA International Fragrance Association”. Archived from the original on 2012-01-06. Retrieved 2012-07-19. “Cropwatch Claims Victory Regarding “26 Allergens” Legislation : Modified from article originally written for Aromaconnection, Feb 2008″ (PDF). Leffingwell.com. Retrieved 2 December 2018.
Minor neurological dysfunction was found in children exposed to the anticoagulants acenocoumarol or phenprocoumon during pregnancy. A group of 306 children were tested at ages 7–15 years to determine subtle neurological effects from anticoagulant exposure. Results showed a dose–response relationship between anticoagulant exposure and minor neurological dysfunction. Overall, a 1.9 (90%) increase in minor neurological dysfunction was observed for children exposed to these anticoagulants, which are collectively referred to as “coumarins.” In conclusion, researchers stated, “The results suggest that coumarins have an influence on the development of the brain which can lead to mild neurologic dysfunctions in children of school age.“Wessling, J. (2001). “Neurological outcome in school-age children after in utero exposure to coumarins”. Early Human Development. 63 (2): 83–95. doi:10.1016/S0378-3782(01)00140-2. PMID 11408097.
Coumarin’s presence in cigarette tobacco caused Brown & Williamson executive Dr. Jeffrey Wigand to contact CBS‘s news show 60 Minutes in 1995, charging that a “form of rat poison” was in the tobacco. He held that from a chemist’s point of view, coumarin is an “immediate precursor” to the rodenticide coumadin. Dr. Wigand later stated that coumarin itself is dangerous, pointing out that the FDA had banned its addition to human food in 1954. Under his later testimony, he would repeatedly classify coumarin as a “lung-specific carcinogen.” In Germany, coumarin is banned as an additive in tobacco. “Jeffrey Wigand : Jeffrey Wigand on 60 Minutes”. Jeffreywigand.com. Retrieved 30 December 2015. “Tobacco On Trial”. Tobacco-on-trial.com. Retrieved 30 December 2015. “Industry Documents Library”. Legacy.library.ucsf.edu. Retrieved 2 December 2018.
Alcoholic beverages sold in the European Union are limited to a maximum of 10 mg/L coumarin by law. Cinnamon flavor is generally cassia bark steam-distilled to concentrate the cinnamaldehyde, for example, to about 93%. Clear cinnamon-flavored alcoholic beverages generally test negative for coumarin, but if whole cassia bark is used to make mulled wine, then coumarin shows up at significant levels. Wang, YH; Avula, B.; Zhao, J.; Smillie, TJ; Nanayakkara, NPD; Khan, IA (2010). “Characterization and Distribution of Coumarin, Cinnamaldehyde and Related Compounds in Cinnamomum spp. by UPLC-UV/MS Combined with PCA”. Planta Medica. 76 (5). doi:10.1055/s-0030-1251793.
Types of phenylpropanoids |
---|
Types of coumarins |
---|
Aspartame
Coumarins + Food
“The rate of absorption of dicoumarol can be increased by food. Two reports describe antagonism of the effects of warfarin by ice cream, and another report attributes an increase in prothrombin time to the use of aspartame. However, the most common food warfarin interaction is that due to foods containing vitamin K”. Saeed AA, Aspartame Sweetener, World Journal of Pharmacy and Pharmaceutical Sciences Volume 9, Issue 2, 195-201 February 2020. DOI:10.20959/wjpps20202-15435 citing K. Baxter, Stockley’s Drug Interactions, Eighth edition, UK, Pharmaceutical Press, 2008.
Coumadin
“Aspartame (Equal, Nutrasweet) is an artificial sweetener that may increase bleeding time (Clinical Pharmacology and Therapeutics, Feb. and May, 1998). Although this interaction is controversial, we would encourage anyone consuming aspartame and taking Coumadin to monitor the impact on bleeding very carefully.” Terry Graedon, The People’s Pharmacy, “Coumadin Interactions Pose Dangers” The People’s Pharmacy, https://www.peoplespharmacy.com/articles/coumadin-intera June 15, 2015
Warfarin
“A very brief report states that a patient taking warfarin had a raised prothrombin time, possibly due to the use of aspartame.” Saeed AA, Aspartame Sweetener, World Journal of Pharmacy and Pharmaceutical Sciences Volume 9, Issue 2, 195-201 February 2020. DOI:10.20959/wjpps20202-15435 citing K. Baxter, Stockley’s Drug Interactions, Eighth edition, UK, Pharmaceutical Press, 2008.
Simultaneous determination of nonnutritive sweeteners in foods by HPLC/ESI-MS
“Target compounds were quantified using a selective ionization recording (SIR) at m/z 178, 397, 377, 293, 641, 312, 162, and 182 to cyclamate, sucralose, neotame, aspartame, stevioside, alitame, acesulfame-K, and saccharin, respectively, with warfarin sodium (SIR m/z 307) being used as an internal standard. The correlation coefficient of the calibration curve was better than 0.998 (n = 6), in the range of 0.05 to 5.00 microg/mL for cyclamate, 0.30 to 30.0 microg/mL for sucralose, 0.10 to 10.0 microg/mL for neotame, 0.20 to 20.0 microg/mL for aspartame, 0.50 to 15.0 microg/mL for stevioside, 0.08 to 8.00 microg/mL for alitame, 0.10 to 15.0 microg/mL for acesulfame-K, and 0.05 to 5.00 microg/mL for saccharin. The limits of detection (LODs) were below 0.10 microg/mL, whereas the limits of quantification (LOQs) were below 0.30 microg/mL. It is concluded that the method has merits such as high sensitivity, specificity, and simplicity versus the those of the other methods reported in the literature.” Yang DJ, Chen B. Simultaneous determination of nonnutritive sweeteners in foods by HPLC/ESI-MS. J Agric Food Chem. 2009 Apr 22;57(8):3022-7. doi: 10.1021/jf803988u. PMID: 19275236.
- An internal standard in analytical chemistry is a chemical substance that is added in a constant amount to samples, the blank and calibration standards in a chemical analysis. This substance can then be used for calibration by plotting the ratio of the analyte signal to the internal standard signal as a function of the analyte concentration of the standards. This is done to correct for the loss of analyte during sample preparation or sample inlet. The internal standard is a compound that is very similar, but not identical to the chemical species of interest in the samples, as the effects of sample preparation should, relative to the amount of each species, be the same for the signal from the internal standard as for the signal(s) from the species of interest in the ideal case. Adding known quantities of analyte(s) of interest is a distinct technique called standard addition, which is performed to correct for matrix effects.
- This ratio for the samples is then used to obtain their analyte concentrations from a calibration curve. The internal standard used needs to provide a signal that is similar to the analyte signal in most ways but sufficiently different so that the two signals are readily distinguishable by the instrument. For example, deuterated chlorobenzene (C6D5Cl) is an internal standard used in the analysis of volatiles on GC-MS because it is similar to Chlorobenzene but does not occur naturally. Norleucine is also a popular internal standard for the analysis of amino acids via GC-MS.
- In NMR spectroscopy, e.g. of the nuclei 1H, 13C and 29Si, frequencies depend on the magnetic field, which is not the same across all experiments. Therefore, frequencies are reported as relative differences to the internal standard tetramethylsilane (TMS). This relative difference to TMS is called chemical shift, and measured in parts per million.
- In practice, the difference between the signals of common solvents and TMS are known, and since modern instruments are capable of detecting the small quantities of protonated solvent present in commercial deuterated solvents no TMS need be added. By specifying the lock solvent to be used, modern spectrometers are able to correctly reference the sample; in effect, the solvent itself serves as the internal standard.
- In chromatography, internal standards are used to determine the concentration of other analytes by calculating response factor. The internal standard selected should be again similar to the analyte and have a similar retention time and similar derivitization. It must be stable and must not interfere with the sample components.
- Skoog, Douglas A. (1998). Principles of Instrumental Analysis: Introduction, pp. 18. Harcourt Brace. ISBN 0-03-002078-6 IUPAC, Compendium of Chemical Terminology, 2nd ed. (the “Gold Book”) (1997). Online corrected version: (2006–) “internal standard”. doi:10.1351/goldbook.I03108