Tetramers and tetrameric protein
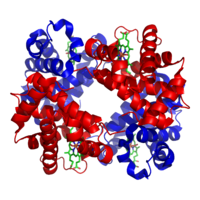
A tetramer is an oligomer formed from four monomers or subunits. The associated property is called tetramery.
An example from inorganic chemistry is titanium methoxide with the empirical formula Ti(OCH3)4, which is tetrameric in solid state and has the molecular formula Ti4(OCH3)16. An example from organic chemistry is kobophenol A, a substance that is formed by combining four molecules of resveratrol.
- Wright, D. A.; Williams, D. A. (1968). “The crystal and molecular structure of titanium tetramethoxide”. Acta Crystallogr. B. 24 (8): 1107–1114. doi:10.1107/S0567740868003766.
- Shu, Na; Zhou, Hong; Hu, Changqi (2006). “Simultaneous determination of the contents of three stilbene oligomers in Caragana sinica collected in different seasons using an improved HPLC method”. Biol. Pharm. Bull. 29 (4): 608–612. doi:10.1248/bpb.29.608. PMID 16595888.
- Li, Liya; Henry, Geneive E.; Seeram, Navindra P. (2009). “Identification and bioactivities of resveratrol oligomers and flavonoids from Carex folliculata seeds”. J. Agric. Food Chem. 57 (16): 7282–7287. doi:10.1021/jf901716j. PMID 19627089.
In biochemistry, it similarly refers to a biomolecule formed of four units, that are the same (homotetramer), i.e. as in Concanavalin A or different (heterotetramer), i.e. as in hemoglobin. Hemoglobin has 4 similar sub-units while immunoglobulins have 2 very different sub-units. The different sub-units may have each their own activity, such as binding biotin in avidin tetramers, or have a common biological property, such as the allosteric binding of oxygen in hemoglobin.
See also
- Cluster chemistry; atomic and molecular clusters
- In chemistry, an atom cluster (or simply cluster) is an ensemble of bound atoms or molecules that is intermediate in size between a simple molecule and a nanoparticle; that is, up to a few nanometers (nm) in diameter. The term microcluster may be used for ensembles with up to couple dozen atoms. Clusters with a definite number and type of atoms in a specific arrangement are often considered a specific chemical compound and are studied as such. For example, fullerene is a cluster of 60 carbon atoms arranged as the vertices of a truncated icosahedron, and decaborane is a cluster of 10 boron atoms forming an incomplete icosahedron, surrounded by 14 hydrogen atoms. The term is most commonly used for ensembles consisting of several atoms of the same element, or of a few different elements, bonded in a three-dimensional arrangement. Transition metals and main group elements form especially robust clusters. Indeed, in some contexts, the term may refer specifically to a metal cluster, whose core atoms are metals and contains at least one metallic bond. In this case, the qualifier poly specifies a cluster with more than one metal atom, and heteronuclear specifies a cluster with at least two different metal elements. Naked metal clusters have only metal atoms, as opposed to clusters with outer shell of other elements. The latter may be functional groups such as cyanide or methyl, covalently bonded to the core atoms; or many be ligands attached by coordination bonds, such as carbon monoxide, halides, isocyanides, alkenes, and hydrides. However, the term is also used for ensembles that contain no metals (such as the boranes and carboranes) and whose core atoms are held together by covalent or ionic bonds. It is also used for ensembles of atoms or molecules held together by Van der Waals or hydrogen bonds, as in water clusters. Clusters may play an important role in phase transitions such as precipitation from solutions, condensation and evaporation of liquids and solids, freezing and melting, and adsorption to other materials.[citation needed]
- Inorganic Chemistry Huheey, JE, 3rd ed. Harper and Row, New York
- Mingos, D. M. P.; Wales, D. J. (1990). Introduction to cluster chemistry. Englewood Cliffs, N.J: Prentice Hall. ISBN 0134743059.
- In chemistry, an atom cluster (or simply cluster) is an ensemble of bound atoms or molecules that is intermediate in size between a simple molecule and a nanoparticle; that is, up to a few nanometers (nm) in diameter. The term microcluster may be used for ensembles with up to couple dozen atoms. Clusters with a definite number and type of atoms in a specific arrangement are often considered a specific chemical compound and are studied as such. For example, fullerene is a cluster of 60 carbon atoms arranged as the vertices of a truncated icosahedron, and decaborane is a cluster of 10 boron atoms forming an incomplete icosahedron, surrounded by 14 hydrogen atoms. The term is most commonly used for ensembles consisting of several atoms of the same element, or of a few different elements, bonded in a three-dimensional arrangement. Transition metals and main group elements form especially robust clusters. Indeed, in some contexts, the term may refer specifically to a metal cluster, whose core atoms are metals and contains at least one metallic bond. In this case, the qualifier poly specifies a cluster with more than one metal atom, and heteronuclear specifies a cluster with at least two different metal elements. Naked metal clusters have only metal atoms, as opposed to clusters with outer shell of other elements. The latter may be functional groups such as cyanide or methyl, covalently bonded to the core atoms; or many be ligands attached by coordination bonds, such as carbon monoxide, halides, isocyanides, alkenes, and hydrides. However, the term is also used for ensembles that contain no metals (such as the boranes and carboranes) and whose core atoms are held together by covalent or ionic bonds. It is also used for ensembles of atoms or molecules held together by Van der Waals or hydrogen bonds, as in water clusters. Clusters may play an important role in phase transitions such as precipitation from solutions, condensation and evaporation of liquids and solids, freezing and melting, and adsorption to other materials.[citation needed]
- Tetramerium, a genus of plants belonging to the family Acanthaceae
- Tetramery (botany), having four parts in a distinct whorl of a plant structure
- Merosity (from the greek “méros,” which means “having parts”) refers to the number of component parts in a distinct whorl of a plant structure.[1] The term is most commonly used in the context of a flower where it refers to the number of sepals in a whorl of the calyx, the number of petals in a whorl of the corolla, the number of stamens in a whorl of the androecium, or the number of carpels in a whorl of the gynoecium. The term may also be used to refer to the number of leaves in a leaf whorl. In nature, five or three parts per whorl have the highest frequency of occurrence, but four or two parts per whorl are not uncommon. Two consecutive whorls of dimerous petals are often mistaken for tetramerous petals. If all of the whorls in a given floral arrangement have the same merosity, the flower is said to be isomerous (equal parts), otherwise the flower is anisomerous (unequal parts). For example, Trillium is isomerous since all whorls are trimerous (one whorl of three sepals, zero or one whorl of three petals, two whorls of three stamens each, and one whorl of three carpels). Trillium also has one whorl of three leaves.
- Ronse Decraene, L. P.; Smets, E. F. (1994). “Merosity in flowers: definition, origin, and taxonomic significance”. Plant Systematics and Evolution. 191 (1–2): 83–104. doi:10.1007/BF00985344. Retrieved 22 November 2019.
- Eckel, P. M. “A Grammatical Dictionary of Botanical Latin”. Retrieved 22 November 2019.
- Merosity (from the greek “méros,” which means “having parts”) refers to the number of component parts in a distinct whorl of a plant structure.[1] The term is most commonly used in the context of a flower where it refers to the number of sepals in a whorl of the calyx, the number of petals in a whorl of the corolla, the number of stamens in a whorl of the androecium, or the number of carpels in a whorl of the gynoecium. The term may also be used to refer to the number of leaves in a leaf whorl. In nature, five or three parts per whorl have the highest frequency of occurrence, but four or two parts per whorl are not uncommon. Two consecutive whorls of dimerous petals are often mistaken for tetramerous petals. If all of the whorls in a given floral arrangement have the same merosity, the flower is said to be isomerous (equal parts), otherwise the flower is anisomerous (unequal parts). For example, Trillium is isomerous since all whorls are trimerous (one whorl of three sepals, zero or one whorl of three petals, two whorls of three stamens each, and one whorl of three carpels). Trillium also has one whorl of three leaves.
- Tetrameric protein
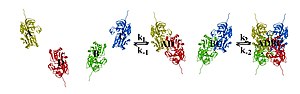
A tetrameric protein is a protein with a quaternary structure of four subunits (tetrameric). Homotetramers have four identical subunits (such as glutathione S-transferase), and heterotetramers are complexes of different subunits. A tetramer can be assembled as dimer of dimers with two homodimer subunits (such as sorbitol dehydrogenase), or two heterodimer subunits (such as hemoglobin).
Subunit interactions in tetramers
The interactions between subunits forming a tetramer is primarily determined by non covalent interaction. Hydrophobic effects, hydrogen bonds and electrostatic interactions are the primary sources for this binding process between subunits. For homotetrameric proteins such as sorbitol dehydrogenase (SDH), the structure is believed to have evolved going from a monomeric to a dimeric and finally a tetrameric structure in evolution. The binding process in SDH and many other tetrameric enzymes can be described by the gain in free energy which can be determined from the rate of association and dissociation. The above image shows the assembly of the four subunits (A,B,C and D) in SDH.
- Hellgren M, Kaiser C, de Haij S, Norberg A, Höög JO (December 2007). “A hydrogen-bonding network in mammalian sorbitol dehydrogenase stabilizes the tetrameric state and is essential for the catalytic power”. Cellular and Molecular Life Sciences. 64 (23): 3129–3138. doi:10.1007/s00018-007-7318-1. PMID 17952367. S2CID 22090973.
Hydrogen bonds between subunits
Hydrogen bonding networks between subunits has been shown to be important for the stability of the tetrameric quaternary protein structure. For example, a study of SDH which used diverse methods such as protein sequence alignments, structural comparisons, energy calculations, gel filtration experiments and enzyme kinetics experiments, could reveal an important hydrogen bonding network which stabilizes the tetrameric quaternary structure in mammalian SDH.
- Hellgren M, Kaiser C, de Haij S, Norberg A, Höög JO (December 2007). “A hydrogen-bonding network in mammalian sorbitol dehydrogenase stabilizes the tetrameric state and is essential for the catalytic power”. Cellular and Molecular Life Sciences. 64 (23): 3129–3138. doi:10.1007/s00018-007-7318-1. PMID 17952367. S2CID 22090973.
Tetramers in immunology
In immunology, MHC tetramers can be used in tetramer assays, to quantify numbers of antigen-specific T cells (especially CD8+ T cells). MHC tetramers are based on recombinant class I molecules that, through the action of bacterial BirA, have been biotinylated. These molecules are folded with the peptide of interest and β2M and tetramerized by a fluorescently labeled streptavidin. (Streptavidin binds to four biotins per molecule.) This tetramer reagent will specifically label T cells that express T cell receptors that are specific for a given peptide-MHC complex. For example, a Kb/FAPGNYPAL tetramer will specifically bind to Sendai virus specific cytotoxic T cell in a C57BL/6 mouse. Antigen specific responses can be measured as CD8+, tetramer+ T cells as a fraction of all CD8+ lymphocytes.
The reason for using a tetramer, as opposed to a single labeled MHC class I molecule is that the tetrahedral tetramers can bind to three TCRs at once, allowing specific binding in spite of the low (1 micromolar) affinity of the typical class I-peptide-TCR interaction. MHC class II tetramers can also be made, although these are more difficult to work with practically.
- Dolton G, Tungatt K, Lloyd A, Bianchi V, Theaker SM, Trimby A, et al. (September 2015). “More tricks with tetramers: a practical guide to staining T cells with peptide-MHC multimers”. Immunology. 146 (1): 11–22. doi:10.1111/imm.12499. PMC 4552497. PMID 26076649.
MHC Class II Wikipedia full page
MHC Class II molecules are a class of major histocompatibility complex (MHC) molecules normally found only on professional antigen-presenting cells such as dendritic cells, mononuclear phagocytes, some endothelial cells, thymic epithelial cells, and B cells. These cells are important in initiating immune responses. The antigens presented by class II peptides are derived from extracellular proteins (not cytosolic as in MHC class I). Loading of a MHC class II molecule occurs by phagocytosis; extracellular proteins are endocytosed, digested in lysosomes, and the resulting epitopic peptide fragments are loaded onto MHC class II molecules prior to their migration to the cell surface. In humans, the MHC class II protein complex is encoded by the human leukocyte antigen gene complex (HLA). HLAs corresponding to MHC class II are HLA-DP, HLA-DM, HLA-DOA, HLA-DOB, HLA-DQ, and HLA-DR. Mutations in the HLA gene complex can lead to bare lymphocyte syndrome (BLS), which is a type of MHC class II deficiency. Like MHC class I molecules, class II molecules are also heterodimers, but in this case consist of two homogenous peptides, an α and β chain, both of which are encoded in the MHC. The subdesignation α1, α2, etc. refers to separate domains within the HLA gene; each domain is usually encoded by a different exon within the gene, and some genes have further domains that encode leader sequences, transmembrane sequences, etc. These molecules have both extracellular regions as well as a transmembrane sequence and a cytoplasmic tail. The α1 and β1 regions of the chains come together to make a membrane-distal peptide-binding domain, while the α2 and β2 regions, the remaining extracellular parts of the chains, form a membrane-proximal immunoglobulin-like domain. The antigen binding groove, where the antigen or peptide binds, is made up of two α-helixes walls and β-sheet. Because the antigen-binding groove of MHC class II molecules is open at both ends while the corresponding groove on class I molecules is closed at each end, the antigens presented by MHC class II molecules are longer, generally between 15 and 24 amino acid residues long. These molecules are constitutively expressed in professional, immune antigen-presenting cells, but may also be induced on other cells by interferon γ. They are expressed on the epithelial cells in the thymus and on APCs in the periphery. MHC class II expression is closely regulated in APCs by CIITA, which is the MHC class II transactivator. CIITA is solely expressed on professional APCs; however, non-professional APCs can also regulate CIITA activity and MHC II expression. As mentioned interferon γ (IFN γ ) triggers the expression of CIITA and is also responsible for converting monocytes which are MHC class II negative cells into functional APCs that express MHC class II on their surfaces. MHC class II is also expressed on group 3 innate lymphoid cells.’
See also
- Cross-presentation
- The first evidence of cross-presentation was reported in 1976 by Michael J. Bevan after injection of grafted cells carrying foreign minor histocompatibility (MHC) molecules. This resulted in a CD8+ T cell response induced by antigen-presenting cells of the recipient against the foreign MHC cells. Because of this, Bevan implied that these antigen presenting cells must have engulfed and cross presented these foreign MHC cells to host cytotoxic CD8+ cells, thus triggering an adaptive immune response against the grafted tissue. This observation was termed “cross-priming”. Later, there had been much controversy about cross-presentation, which now is believed to have been due to particularities and limitations of some experimental systems used.
- Gutiérrez-Martínez, Enric; Planès, Remi; Anselmi, Giorgio; Reynolds, Matthew; Menezes, Shinelle; Adiko, Aimé Cézaire; Saveanu, Loredana; Guermonprez, Pierre (2015). “Cross-Presentation of Cell-Associated Antigens by MHC Class I in Dendritic Cell Subsets”. Frontiers in Immunology. 6: 363. doi:10.3389/fimmu.2015.00363. ISSN 1664-3224. PMC 4505393. PMID 26236315.
- Bevan, MJ (1976). “Cross-priming for a secondary cytotoxic response to minor H antigens with H-2 congenic cells which do not cross-react in the cytotoxic assay”. J. Exp. Med. 143 (5): 1283–8. doi:10.1084/jem.143.5.1283. PMC 2190184. PMID 1083422.
- Wolkers, MC; Brouwenstijn, N; Bakker, AH; Toebes, M; Schumacher, TN (2004). “Antigen bias in T cell cross-priming”. Science. 304 (5675): 1314–7. Bibcode:2004Sci…304.1314W. doi:10.1126/science.1096268. PMID 15166378. S2CID 6681264.
- The first evidence of cross-presentation was reported in 1976 by Michael J. Bevan after injection of grafted cells carrying foreign minor histocompatibility (MHC) molecules. This resulted in a CD8+ T cell response induced by antigen-presenting cells of the recipient against the foreign MHC cells. Because of this, Bevan implied that these antigen presenting cells must have engulfed and cross presented these foreign MHC cells to host cytotoxic CD8+ cells, thus triggering an adaptive immune response against the grafted tissue. This observation was termed “cross-priming”. Later, there had been much controversy about cross-presentation, which now is believed to have been due to particularities and limitations of some experimental systems used.
- Bare lymphocyte syndrome
- Bare lymphocyte syndrome is a condition caused by mutations in certain genes of the major histocompatibility complex or involved with the processing and presentation of MHC molecules. It is a form of severe combined immunodeficiency.
- DeSandro AM, Nagarajan UM, Boss JM (September 2000). “Associations and interactions between bare lymphocyte syndrome factors”. Mol. Cell. Biol. 20 (17): 6587–99. doi:10.1128/MCB.20.17.6587-6599.2000. PMC 86141. PMID 10938133.
- Bare lymphocyte syndrome is a condition caused by mutations in certain genes of the major histocompatibility complex or involved with the processing and presentation of MHC molecules. It is a form of severe combined immunodeficiency.
- Histocompatibility”. Archived from the original on 2008-12-26. Retrieved 2009-01-21.
- Jones EY, Fugger L, Strominger JL, Siebold C (April 2006). “MHC class II proteins and disease: a structural perspective”. Nature Reviews. Immunology. 6 (4): 271–82. doi:10.1038/nri1805. PMID 16557259. S2CID 131777.
- Ting JP, Trowsdale J (April 2002). “Genetic control of MHC class II expression”. Cell. 109 Suppl (2): S21-33. doi:10.1016/s0092-8674(02)00696-7. PMID 11983150.
- Roche PA, Furuta K (April 2015). “The ins and outs of MHC class II-mediated antigen processing and presentation”. Nature Reviews. Immunology. 15 (4): 203–16. doi:10.1038/nri3818. PMC 6314495. PMID 25720354.
Homotetramers and heterotetramers
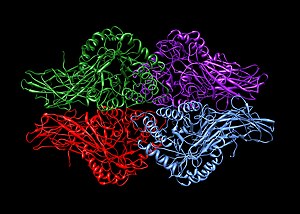
A homotetramer is a protein complex made up of four identical subunits which are associated but not covalently bound. Conversely, a heterotetramer is a 4-subunit complex where one or more subunits differ.
- “GO term: protein homotetramerization”. YeastGenome. Archived from the original on 27 September 2011. Retrieved 14 May 2011.
- “GO term: protein heterotetramerization”. YeastGenome. Archived from the original on 27 September 2011. Retrieved 14 May 2011.
Examples of homotetramers include:
- enzymes like beta-glucuronidase (pictured)
- export factors such as SecB from Escherichia coli
- magnesium ion transporters such as CorA.
- lectins such as Concanavalin A
- IMPDH and IMPDH2
- Watanabe M, Blobel G (April 1989). “Cytosolic factor purified from Escherichia coli is necessary and sufficient for the export of a preprotein and is a homotetramer of SecB”. Proceedings of the National Academy of Sciences of the United States of America. 86 (8): 2728–2732. Bibcode:1989PNAS…86.2728W. doi:10.1073/pnas.86.8.2728. PMC 286991. PMID 2649892.
- Warren MA, Kucharski LM, Veenstra A, Shi L, Grulich PF, Maguire ME (July 2004). “The CorA Mg2+ transporter is a homotetramer”. Journal of Bacteriology. 186 (14): 4605–4612. doi:10.1128/JB.186.14.4605-4612.2004. PMC 438605. PMID 15231793.
Examples of heterotetramers include haemoglobin (pictured), the NMDA receptor, some aquaporins, some AMPA receptors, as well as some enzymes.
- Neely JD, Christensen BM, Nielsen S, Agre P (August 1999). “Heterotetrameric composition of aquaporin-4 water channels”. Biochemistry. 38 (34): 11156–11163. doi:10.1021/bi990941s. PMID 10460172.
- Chang TH, Hsieh FL, Ko TP, Teng KH, Liang PH, Wang AH (February 2010). “Structure of a heterotetrameric geranyl pyrophosphate synthase from mint (Mentha piperita) reveals intersubunit regulation”. The Plant Cell. 22 (2): 454–467. doi:10.1105/tpc.109.071738. PMC 2845413. PMID 20139160.
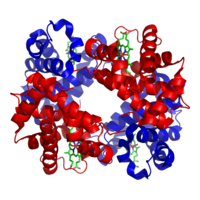
Purification of heterotetramers
Ion-exchange chromatography is useful for isolating specific heterotetrameric protein assemblies, allowing purification of specific complexes according to both the number and the position of charged peptide tags. Nickel affinity chromatography may also be employed for heterotetramer purification.
- Sakash JB, Kantrowitz ER (September 2000). “The contribution of individual interchain interactions to the stabilization of the T and R states of Escherichia coli aspartate transcarbamoylase”. The Journal of Biological Chemistry. 275 (37): 28701–28707. doi:10.1074/jbc.M005079200. PMID 10875936.
- Fairhead M, Krndija D, Lowe ED, Howarth M (January 2014). “Plug-and-play pairing via defined divalent streptavidins”. Journal of Molecular Biology. 426 (1): 199–214. doi:10.1016/j.jmb.2013.09.016. PMC 4047826. PMID 24056174.
- Howarth M, Chinnapen DJ, Gerrow K, Dorrestein PC, Grandy MR, Kelleher NL, et al. (April 2006). “A monovalent streptavidin with a single femtomolar biotin binding site”. Nature Methods. 3 (4): 267–273. doi:10.1038/nmeth861. PMC 2576293. PMID 16554831.
Intragenic complementation
Multiple copies of a polypeptide encoded by a gene often can form an aggregate referred to as a multimer. When a multimer is formed from polypeptides produced by two different mutant alleles of a particular gene, the mixed multimer may exhibit greater functional activity than the unmixed multimers formed by each of the mutants alone. When a mixed multimer displays increased functionality relative to the unmixed multimers, the phenomenon is referred to as intragenic complementation. In humans, argininosuccinate lyase (ASL) is a homotetrameric enzyme that can undergo intragenic complementation. An ASL disorder in humans can arise from mutations in the ASL gene, particularly mutations that affect the active site of the tetrameric enzyme. ASL disorder is associated with considerable clinical and genetic heterogeneity which is considered to reflect the extensive intragenic complementation occurring among different individual patients.
- Turner MA, Simpson A, McInnes RR, Howell PL (August 1997). “Human argininosuccinate lyase: a structural basis for intragenic complementation”. Proceedings of the National Academy of Sciences of the United States of America. 94 (17): 9063–9068. Bibcode:1997PNAS…94.9063T. doi:10.1073/pnas.94.17.9063. PMC 23030. PMID 9256435.
- Yu B, Howell PL (October 2000). “Intragenic complementation and the structure and function of argininosuccinate lyase”. Cellular and Molecular Life Sciences. 57 (11): 1637–1651. doi:10.1007/PL00000646. PMID 11092456. S2CID 1254964.
- Yu B, Thompson GD, Yip P, Howell PL, Davidson AR (December 2001). “Mechanisms for intragenic complementation at the human argininosuccinate lyase locus”. Biochemistry. 40 (51): 15581–15590. doi:10.1021/bi011526e. PMID 11747433
References
- Hellgren M, Kaiser C, de Haij S, Norberg A, Höög JO (December 2007). “A hydrogen-bonding network in mammalian sorbitol dehydrogenase stabilizes the tetrameric state and is essential for the catalytic power”. Cellular and Molecular Life Sciences. 64 (23): 3129–3138. doi:10.1007/s00018-007-7318-1. PMID 17952367. S2CID 22090973.
- Dolton G, Tungatt K, Lloyd A, Bianchi V, Theaker SM, Trimby A, et al. (September 2015). “More tricks with tetramers: a practical guide to staining T cells with peptide-MHC multimers”. Immunology. 146 (1): 11–22. doi:10.1111/imm.12499. PMC 4552497. PMID 26076649.
- “GO term: protein homotetramerization”. YeastGenome. Archived from the original on 27 September 2011. Retrieved 14 May 2011.
- “GO term: protein heterotetramerization”. YeastGenome. Archived from the original on 27 September 2011. Retrieved 14 May 2011.
- Watanabe M, Blobel G (April 1989). “Cytosolic factor purified from Escherichia coli is necessary and sufficient for the export of a preprotein and is a homotetramer of SecB”. Proceedings of the National Academy of Sciences of the United States of America. 86 (8): 2728–2732. Bibcode:1989PNAS…86.2728W. doi:10.1073/pnas.86.8.2728. PMC 286991. PMID 2649892.
- Warren MA, Kucharski LM, Veenstra A, Shi L, Grulich PF, Maguire ME (July 2004). “The CorA Mg2+ transporter is a homotetramer”. Journal of Bacteriology. 186 (14): 4605–4612. doi:10.1128/JB.186.14.4605-4612.2004. PMC 438605. PMID 15231793.
- Neely JD, Christensen BM, Nielsen S, Agre P (August 1999). “Heterotetrameric composition of aquaporin-4 water channels”. Biochemistry. 38 (34): 11156–11163. doi:10.1021/bi990941s. PMID 10460172.
- Chang TH, Hsieh FL, Ko TP, Teng KH, Liang PH, Wang AH (February 2010). “Structure of a heterotetrameric geranyl pyrophosphate synthase from mint (Mentha piperita) reveals intersubunit regulation”. The Plant Cell. 22 (2): 454–467. doi:10.1105/tpc.109.071738. PMC 2845413. PMID 20139160.
- Sakash JB, Kantrowitz ER (September 2000). “The contribution of individual interchain interactions to the stabilization of the T and R states of Escherichia coli aspartate transcarbamoylase”. The Journal of Biological Chemistry. 275 (37): 28701–28707. doi:10.1074/jbc.M005079200. PMID 10875936.
- Fairhead M, Krndija D, Lowe ED, Howarth M (January 2014). “Plug-and-play pairing via defined divalent streptavidins”. Journal of Molecular Biology. 426 (1): 199–214. doi:10.1016/j.jmb.2013.09.016. PMC 4047826. PMID 24056174.
- Howarth M, Chinnapen DJ, Gerrow K, Dorrestein PC, Grandy MR, Kelleher NL, et al. (April 2006). “A monovalent streptavidin with a single femtomolar biotin binding site”. Nature Methods. 3 (4): 267–273. doi:10.1038/nmeth861. PMC 2576293. PMID 16554831.
- Turner MA, Simpson A, McInnes RR, Howell PL (August 1997). “Human argininosuccinate lyase: a structural basis for intragenic complementation”. Proceedings of the National Academy of Sciences of the United States of America. 94 (17): 9063–9068. Bibcode:1997PNAS…94.9063T. doi:10.1073/pnas.94.17.9063. PMC 23030. PMID 9256435.
- Yu B, Howell PL (October 2000). “Intragenic complementation and the structure and function of argininosuccinate lyase”. Cellular and Molecular Life Sciences. 57 (11): 1637–1651. doi:10.1007/PL00000646. PMID 11092456. S2CID 1254964.
- Yu B, Thompson GD, Yip P, Howell PL, Davidson AR (December 2001). “Mechanisms for intragenic complementation at the human argininosuccinate lyase locus”. Biochemistry. 40 (51): 15581–15590. doi:10.1021/bi011526e. PMID 11747433.